25.4 Physiology of Urine Formation: Glomerular Filtration
Learning Objectives
By the end of this section, you will be able to:
- Describe glomerular filtration, including the hydrostatic and colloid osmotic forces that favor and oppose filtration
- Describe glomerular filtration rate (GFR) and net filtration pressure (NFP)
- Predict specific factors that will increase or decrease GFR
- Explain the mechanisms that control renal blood flow to the glomerulus.
- Explain how the kidney filters the blood to produce urine.
- Describe the myogenic and tubuloglomerular feedback mechanisms and explain how they affect urine volume and composition
- Describe the extrinsic mechanisms for controlling GFR
Glomerular Filtration
Filtrate is produced by the glomerulus when the hydrostatic pressure produced by the heart pushes water and solutes through the filtration membrane. Glomerular filtration is a passive process as cellular energy is not used at the filtration membrane to produce filtrate. Recall that the filtration membrane lies between the blood in the glomerulus and the filtrate in the Bowman’s (glomerular) capsule and this filtration membrane is highly fenestrated allowing the passage of small molecules such as water, sodium, glucose, etc.
The volume of filtrate formed by both kidneys per minute is termed glomerular filtration rate (GFR). Approximately 20% of your cardiac output is filtered by your kidneys per minute under resting conditions. The work of the kidneys produces about 125 mL/min filtrate in men (range of 90 to 140 mL/min) and 105 mL/min filtrate in women (range of 80 to 125 mL/min). This amount equates to a volume of about 180 L/day in men and 150 L/day in women. However, 99% of this filtrate is returned to the circulation through reabsorption resulting in only about 1–2 liters of urine per day.
GFR is influenced by multiple factors, like those seen at tissue capillary beds (see chapter 19). Recall that filtration occurs as pressure forces fluid and solutes through a semipermeable barrier with the solute movement constrained by particle size. Hydrostatic pressure is the pressure produced by a fluid against a surface. The blood inside the glomerulus creates glomerular hydrostatic pressure which forces fluid out of the glomerulus into the glomerular capsule. The fluid in the glomerular capsule creates pressure pushing fluid out of the glomerular capsule back into the glomerulus, opposing the glomerular hydrostatic pressure. This is the capsular hydrostatic pressure. These fluids exert pressures in opposing directions. Net fluid movement will be in the direction of the lower pressure. However, the concentration of the solutes in the fluids affects net movement of fluid as well.
Water moves across a membrane from areas of high water concentration (low dissolved solute concentration) to areas of low water concentration (high dissolved solute concentration) through the process of osmosis. The concentration of plasma solutes in the glomerulus is greater than the concentration of the filtrate in the glomerular capsule since the filtration membrane limits the size of particles crossing the membrane. Most proteins cannot pass into the filtrate resulting in water’s movement out of the capsule towards the glomerulus. This pressure acting to draw water into the glomerulus is called blood colloid osmotic pressure. The absence of proteins in the glomerular space (the lumen within the glomerular capsule) results in a capsular osmotic pressure near zero.
Glomerular filtration occurs when glomerular (blood) hydrostatic pressure exceeds the hydrostatic pressure of the glomerular capsule and the blood colloid osmotic pressure. The sum of all of the influences, both osmotic and hydrostatic, results in a net filtration pressure (NFP). Glomerular hydrostatic pressure is typically about 55 mmHg pushing fluid into the glomerular capsule. This outward pressure is countered by a typical capsular hydrostatic pressure of about 15 mmHg and a blood colloid osmotic pressure of 30 mmHg. To calculate the value of NFP:
NFP = Glomerular blood hydrostatic pressure (GBHP) – [capsular hydrostatic pressure (CHP) + blood colloid osmotic pressure (BCOP)] = 10 mm Hg
That is: NFP = GBHP – [CHP + BCOP] = 10 mm Hg
Or: NFP = 55 – [15 + 30] = 10 mm Hg (Figure 25.4.1).
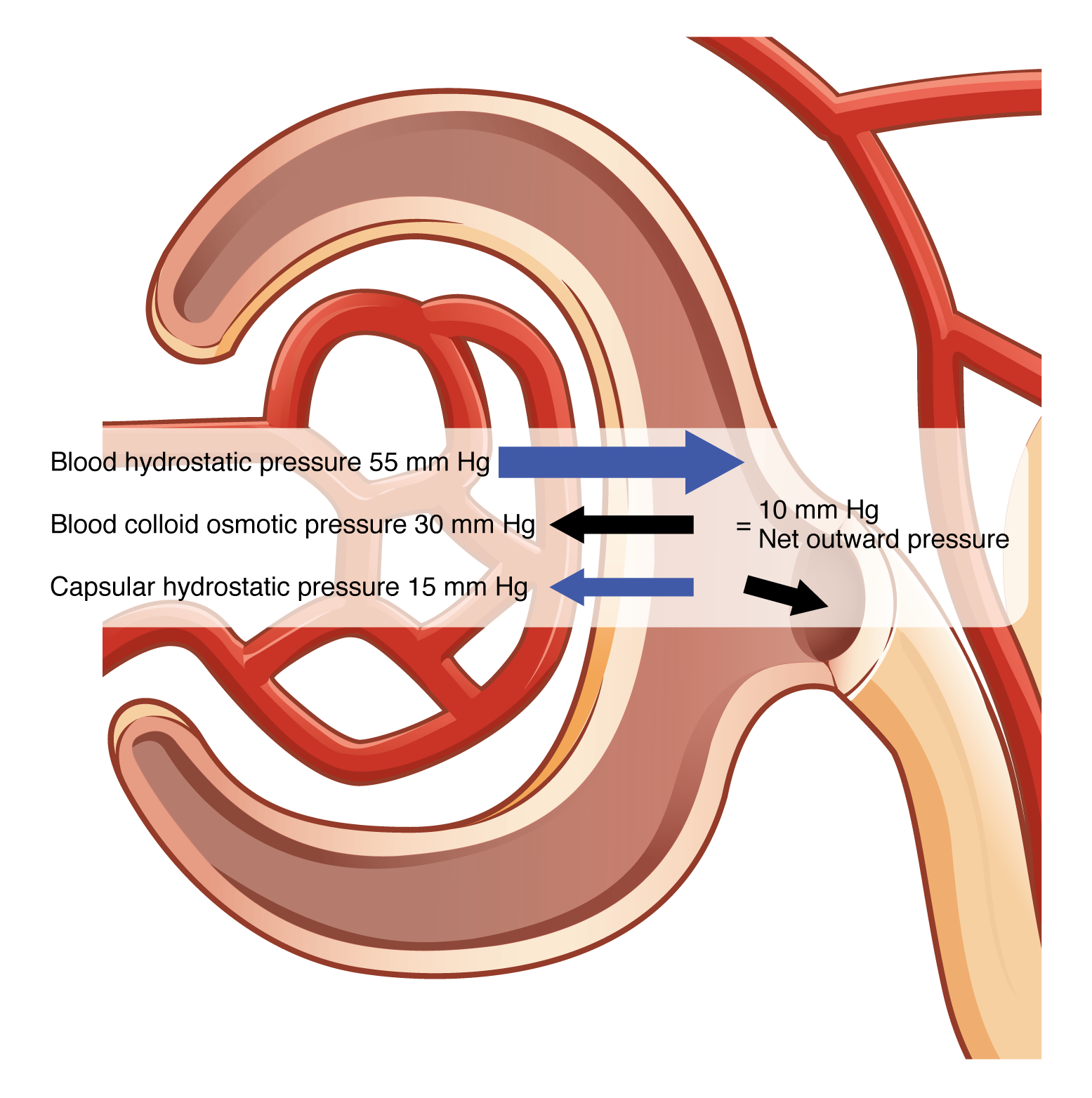
A proper concentration of solutes in the blood is important in maintaining osmotic pressure both in the glomerulus and systemically. There are disorders in which too much protein passes through the filtration slits into the kidney filtrate. This excess protein in the filtrate leads to a deficiency of circulating plasma proteins. Together, blood colloid osmotic pressure decreases, resulting in an increase in urine volume potentially causing dehydration.
As you can see, there is a low net pressure across the filtration membrane. Intuitively, you should realize that minor changes in osmolarity of the blood or changes in capillary blood pressure result in major changes in the amount of filtrate formed at any given point in time. The kidney is able to cope with a wide range of blood pressures. In large part, this is due to the autoregulatory nature of smooth muscle. When you stretch it, it contracts. Thus, when blood pressure goes up, smooth muscle in the afferent arterioles contracts to limit any increase in blood flow and filtration rate. When blood pressure drops, the same capillaries relax to maintain blood flow and filtration rate. The net result is a relatively steady flow of blood into the glomerulus and a relatively steady filtration rate in spite of significant systemic blood pressure changes. Mean arterial blood pressure is calculated by adding 1/3 of the difference between the systolic and diastolic pressures to the diastolic pressure. Therefore, if the blood pressure is 110/80, the difference between systolic and diastolic pressure is 30. One third of this is 10, and when you add this to the diastolic pressure of 80, you arrive at a calculated mean arterial pressure of 90 mm Hg. Therefore, if you use mean arterial pressure for the GBHP in the formula for calculating NFP, you can determine that as long as mean arterial pressure is above approximately 60 mm Hg, the pressure will be adequate to maintain glomerular filtration. Blood pressures below this level will impair renal function and cause systemic disorders that are severe enough to threaten survival. This condition is called shock.
It is vital that the flow of blood through the kidney be at a suitable rate to allow for filtration and yet not too fast to overwhelm the reabsorbing potential of the nephron tubule. This rate determines how much solute is retained or discarded, how much water is retained or discarded, and ultimately, the osmolarity of blood and the blood pressure of the body.
Regulation of GFR
Glomerular filtration has to be carefully and thoroughly controlled because the simple act of filtrate production can have huge impacts on body fluid homeostasis and systemic blood pressure. Due to these two very distinct physiological needs, the body employs two very different mechanisms to regulate GFR. The kidney can control itself locally through intrinsic controls, also called renal autoregulation. These intrinsic control mechanisms maintain filtrate production so that the body can maintain fluid, electrolyte, and acid-base balance and also remove wastes and toxins from the body. There are also control mechanisms that originate outside of the kidney, the nervous and endocrine systems, and are called extrinsic controls. The nervous system and hormones released by the endocrine systems function to control systemic blood pressure by increasing or decreasing GFR to change systemic blood pressure by changing the fluid lost from the body.
Intrinsic Controls: Renal Autoregulation
The kidneys are very effective at regulating the rate of blood flow over a wide range of blood pressures. Your blood pressure will decrease when you are relaxed or sleeping. It will increase when exercising. Yet, despite these changes, the filtration rate through the kidney will change very little. The kidney’s ability to autoregulate can maintain GFR with a MAP of as low as 80 mm Hg to as high as 180 mm Hg. This is due to two internal autoregulatory mechanisms that operate without outside influence: the myogenic mechanism and the tubuloglomerular feedback mechanism.
Arteriole Myogenic Mechanism
The myogenic mechanism regulating blood flow within the kidney depends upon a characteristic shared by most smooth muscle cells of the body. When you stretch a smooth muscle cell, it contracts; when you stop, it relaxes, restoring its resting length. This mechanism works in the afferent arteriole that supplies the glomerulus and can regulate the blood flow into the glomerulus. When blood pressure increases, smooth muscle cells in the wall of the arteriole are stretched and respond by contracting to resist the pressure, resulting in little change in flow. This vasoconstriction of the afferent arteriole acts to reduce excess filtrate formation, maintaining normal NFP and GFR. Reducing the glomerular pressure also functions to protect the fragile capillaries of the glomerulus. When blood pressure drops, the same smooth muscle cells relax to lower resistance, increasing blood flow. The vasodilation of the afferent arteriole acts to increase the declining filtrate formation, bringing NFP and GFR back up to normal levels.
Tubuloglomerular Feedback
The tubuloglomerular feedback mechanism involves the juxtaglomerular (JG) cells, or granular cells, from the juxtaglomerular apparatus (JGA) and a paracrine signaling mechanism utilizing ATP and adenosine. These juxtaglomerular cells are modified, smooth muscle cells lining the afferent arteriole that can contract or relax in response to the paracrine secretions released by the macula densa. This mechanism stimulates either contraction or relaxation of afferent arteriolar smooth muscle cells, which regulates blood flow to the glomerulus (Table 25.8). Recall that the DCT is in intimate contact with the afferent and efferent arterioles of the glomerulus. Specialized macula densa cells in this segment of the tubule respond to changes in the fluid flow rate and Na+ concentration.
As GFR increases, there is less time for NaCl to be reabsorbed in the PCT, resulting in higher osmolarity in the filtrate (hyperosmotic). The increased fluid movement more strongly deflects single nonmotile cilia on macula densa cells. This increased osmolarity of the filtrate, and the greater flow rate within the DCT, activates macula densa cells to respond by releasing ATP and adenosine (a metabolite of ATP). ATP and adenosine act locally as paracrine factors to stimulate the myogenic juxtaglomerular cells of the afferent arteriole to constrict, slowing blood flow into the glomerulus. This vasoconstriction causes less plasma to be filtered, which decreases the glomerular filtration rate (GFR), which gives the tubule more time for NaCl reabsorption. Conversely, when GFR decreases, less NaCl is in the filtrate, and most will be reabsorbed before reaching the macula densa, which will result in decreased ATP and adenosine, allowing the afferent arteriole to dilate and increase GFR. This vasodilation causes more plasma to be filtered, which increase the glomerular filtration rate (GFR), which gives the tubule less time for NaCl reabsorption increasing the amount of NaCl in the filtrate.
Paracrine Mechanisms Controlling Glomerular Filtration Rate (Table 25.8) | |||
---|---|---|---|
Change in GFR | NaCl Absorption | Role of ATP and adenosine/Role of NO | Effect on GFR |
Increased GFR | Tubular NaCl increases | ATP and adenosine increase, causing vasoconstriction | Vasoconstriction slows GFR |
Decreased GFR | Tubular NaCl decreases | ATP and adenosine decrease, causing vasodilation | Vasodilation increases GFR |
Extrinsic Controls: Neural and Hormonal Mechanisms
The extrinsic control mechanisms have an effect on GFR, but their primary function is to maintain systemic blood pressure. While the intrinsic controls functioned to specifically control GFR at the level of the kidneys, the neural and hormonal controls have a broader scope and function to meet the whole body’s needs, not just the needs of the kidneys.
Sympathetic Nerves
The kidneys are innervated by the sympathetic neurons of the autonomic nervous system via the celiac plexus and splanchnic nerves. Reduction of sympathetic stimulation results in vasodilation and increased blood flow through the kidneys during resting conditions. When the frequency of action potentials increases, the arteriolar smooth muscle constricts (vasoconstriction), resulting in diminished glomerular flow, so less filtration occurs. Under conditions of stress, sympathetic nervous activity increases, resulting in the direct vasoconstriction of afferent arterioles (norepinephrine effect) as well as stimulation of the adrenal medulla. The adrenal medulla, in turn, produces a generalized vasoconstriction through the release of epinephrine. This includes vasoconstriction of the afferent arterioles, further reducing the volume of blood flowing through the kidneys. This process redirects blood to other organs with more immediate needs. Under severe stress, such as significant blood loss, the sympathetic nervous system kicks into high gear to keep the blood routed to essential organs and keep the body alive. The strong vasoconstriction required to maintain systemic blood pressure under these severe conditions significantly reduces blood flow to the kidneys and can be damaging to the kidney tissues. If blood pressure falls, the sympathetic nerves will also stimulate the release of renin which we will discuss next.
Renin–Angiotensin–Aldosterone Mechanism
Recall that renin is an enzyme that is produced by the granular cells of the afferent arteriole at the JGA. It enzymatically converts angiotensinogen (made by the liver, freely circulating) into angiotensin I. Its release is stimulated by paracrine signals from the JGA in response to decreased extracellular fluid volume.
Angiotensin-converting enzyme (ACE) enzymatically converts inactive angiotensin I into active angiotensin II. ACE is not a hormone but it is functionally important in regulating systemic blood pressure and kidney function. It is produced in the lungs but binds to the surfaces of endothelial cells in the afferent arterioles and glomerulus. ACE is important in increasing blood pressure and this is why people with high blood pressure are sometimes prescribed ACE inhibitors to lower their blood pressure.
Angiotensin II is a potent vasoconstrictor that plays an immediate role in the regulation of blood pressure. It acts systemically to cause vasoconstriction as well as constriction of both the afferent and efferent arterioles of the glomerulus. Under the influence of Angiotensin II, the efferent arteriole constricts more strongly than the afferent arteriole, increasing GFR. In instances of blood loss or dehydration, Angiotensin II reduces both GFR and renal blood flow, thereby limiting fluid loss and preserving blood volume. Its release is usually stimulated by decreases in blood pressure, and so the preservation of adequate blood pressure is its primary role.
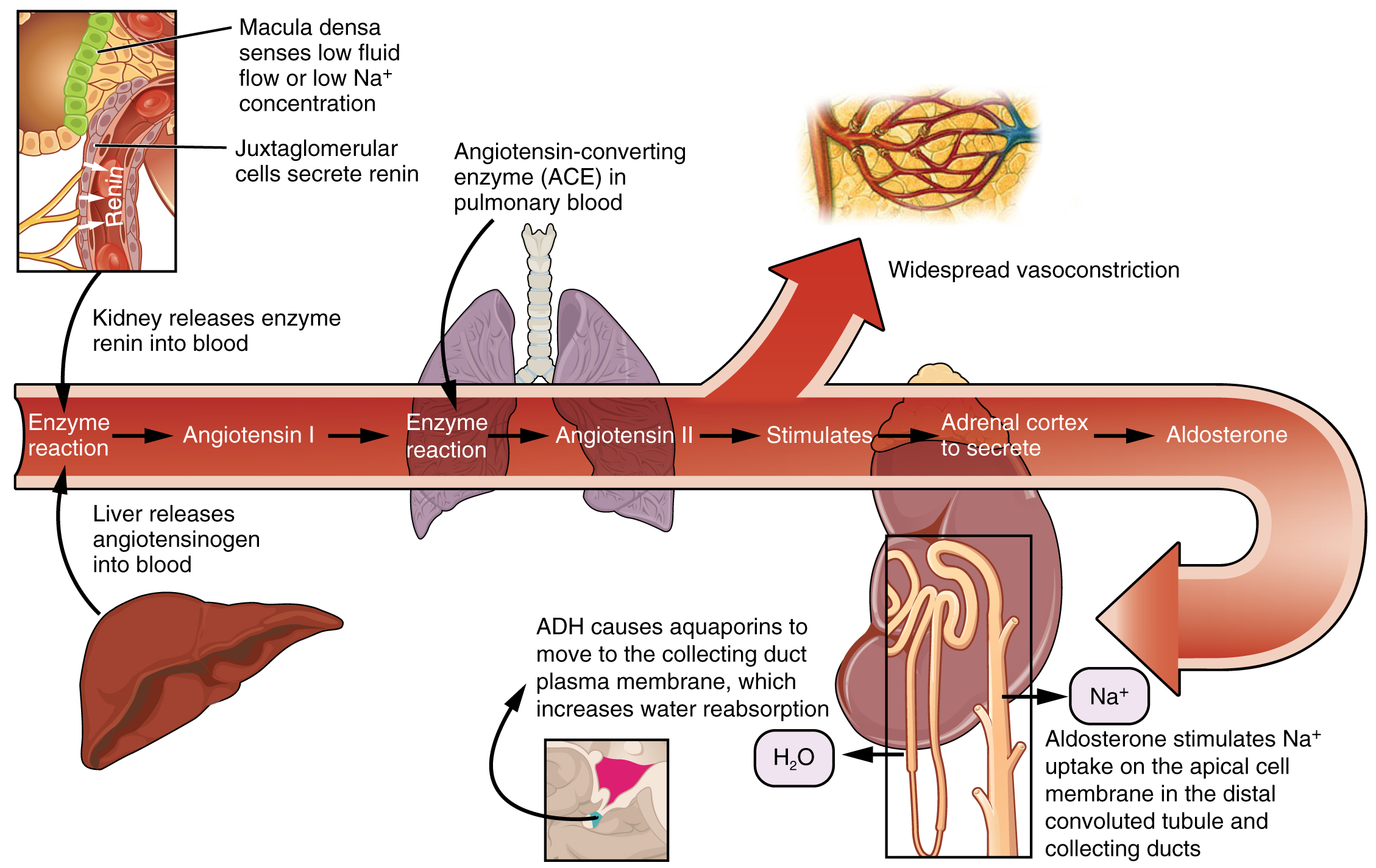
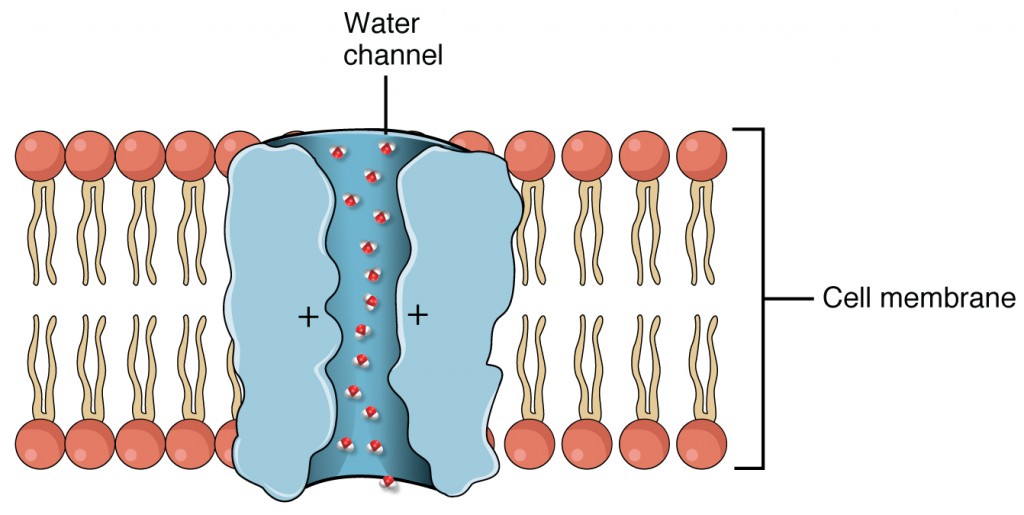
Chapter Review
The GFR is influenced by hydrostatic pressure and colloid osmotic pressure. Under normal circumstances, hydrostatic pressure is significantly greater and filtration occurs.
The kidneys are innervated by sympathetic nerves of the autonomic nervous system. Sympathetic nervous activity decreases blood flow to the kidney, making more blood available to other areas of the body during times of stress. The arteriolar myogenic mechanism maintains a steady blood flow by causing arteriolar smooth muscle to contract when blood pressure increases and causing it to relax when blood pressure decreases. Tubuloglomerular feedback involves paracrine signaling at the JGA to cause vasoconstriction or vasodilation to maintain a steady rate of blood flow.
Review Questions
1. Vasodilation of blood vessels to the kidneys is due to ________.
- more frequent action potentials
- less frequent action potentials
2. When blood pressure increases, blood vessels supplying the kidney will ________ to mount a steady rate of filtration.
- contract
- relax
3. ________ pressure must be greater on the capillary side of the filtration membrane to achieve filtration.
- Osmotic
- Hydrostatic
4. Systemic blood pressure must stay above 60 so that the proper amount of filtration occurs.
- true
- false
Critical Thinking Questions
1. Explain what happens to Na+ concentration in the nephron when GFR increases.
2. If you want the kidney to excrete more Na+ in the urine, what do you want the blood flow to do?
3. Give the formula for net filtration pressure.
Glossary
- glomerular filtration rate (GFR)
- rate of renal filtration
- intercalated cell
- specialized cell of the collecting ducts that secrete or absorb acid or bicarbonate; important in acid–base balance
- myogenic mechanism
- mechanism by which smooth muscle responds to stretch by contracting; an increase in blood pressure causes vasoconstriction and a decrease in blood pressure causes vasodilation so that blood flow downstream remains steady
- net filtration pressure (NFP)
- pressure of fluid across the glomerulus; calculated by taking the hydrostatic pressure of the capillary and subtracting the colloid osmotic pressure of the blood and the hydrostatic pressure of Bowman’s capsule
- principal cell
- found in collecting ducts and possess channels for the recovery or loss of sodium and potassium; under the control of aldosterone; also have aquaporin channels under ADH control to regulate recovery of water
- systemic edema
- increased fluid retention in the interstitial spaces and cells of the body; can be seen as swelling over large areas of the body, particularly the lower extremities
- tubuloglomerular feedback
- feedback mechanism involving the JGA; macula densa cells monitor Na+ concentration in the terminal portion of the ascending loop of Henle and act to cause vasoconstriction or vasodilation of afferent and efferent arterioles to alter GFR
Solutions
Answers for Review Questions
- B
- A
Answers for Critical Thinking Questions
- Sodium concentration in the filtrate increases when GFR increases; it will decrease when GFR decreases.
- To excrete more Na+ in the urine, increase the flow rate.
- Net filtration pressure (NFP) = glomerular blood hydrostatic pressure (GBHP) – [capsular hydrostatic pressure (CHP) + blood colloid osmotic pressure (BCOP).
This work, Anatomy & Physiology, is adapted from Anatomy & Physiology by OpenStax, licensed under CC BY. This edition, with revised content and artwork, is licensed under CC BY-SA except where otherwise noted.
Images, from Anatomy & Physiology by OpenStax, are licensed under CC BY except where otherwise noted.
Access the original for free at https://openstax.org/books/anatomy-and-physiology/pages/1-introduction.