15.3 Hearing
Learning Objectives
By the end of this section, you will be able to:
- Describe the structures responsible for the special senses of hearing.
- Describe the means of mechanoreception for hearing
Audition (Hearing)
Hearing, or audition, is the transduction of sound waves into a neural signal that is made possible by the structures of the ear (Figure 15.3.1). The large, fleshy structure on the lateral aspect of the head is known as the auricle. Some sources will also refer to this structure as the pinna, though that term is more appropriate for a structure that can be moved, such as the external ear of a cat. The C-shaped curves of the auricle direct sound waves toward the auditory canal. The canal enters the skull through the external auditory meatus of the temporal bone. At the end of the auditory canal is the tympanic membrane, or ear drum, which vibrates after it is struck by sound waves. The auricle, ear canal, and tympanic membrane are often referred to as the external ear. The middle ear consists of a space spanned by three small bones called the ossicles. The three ossicles are the malleus, incus, and stapes, which are Latin names that roughly translate to hammer, anvil, and stirrup. The malleus is attached to the tympanic membrane and articulates with the incus. The incus, in turn, articulates with the stapes. The stapes is then attached to the inner ear, where the sound waves will be transduced into a neural signal. The middle ear is connected to the pharynx through the Eustachian tube, which helps equilibrate air pressure across the tympanic membrane. The tube is normally closed but will pop open when the muscles of the pharynx contract during swallowing or yawning.
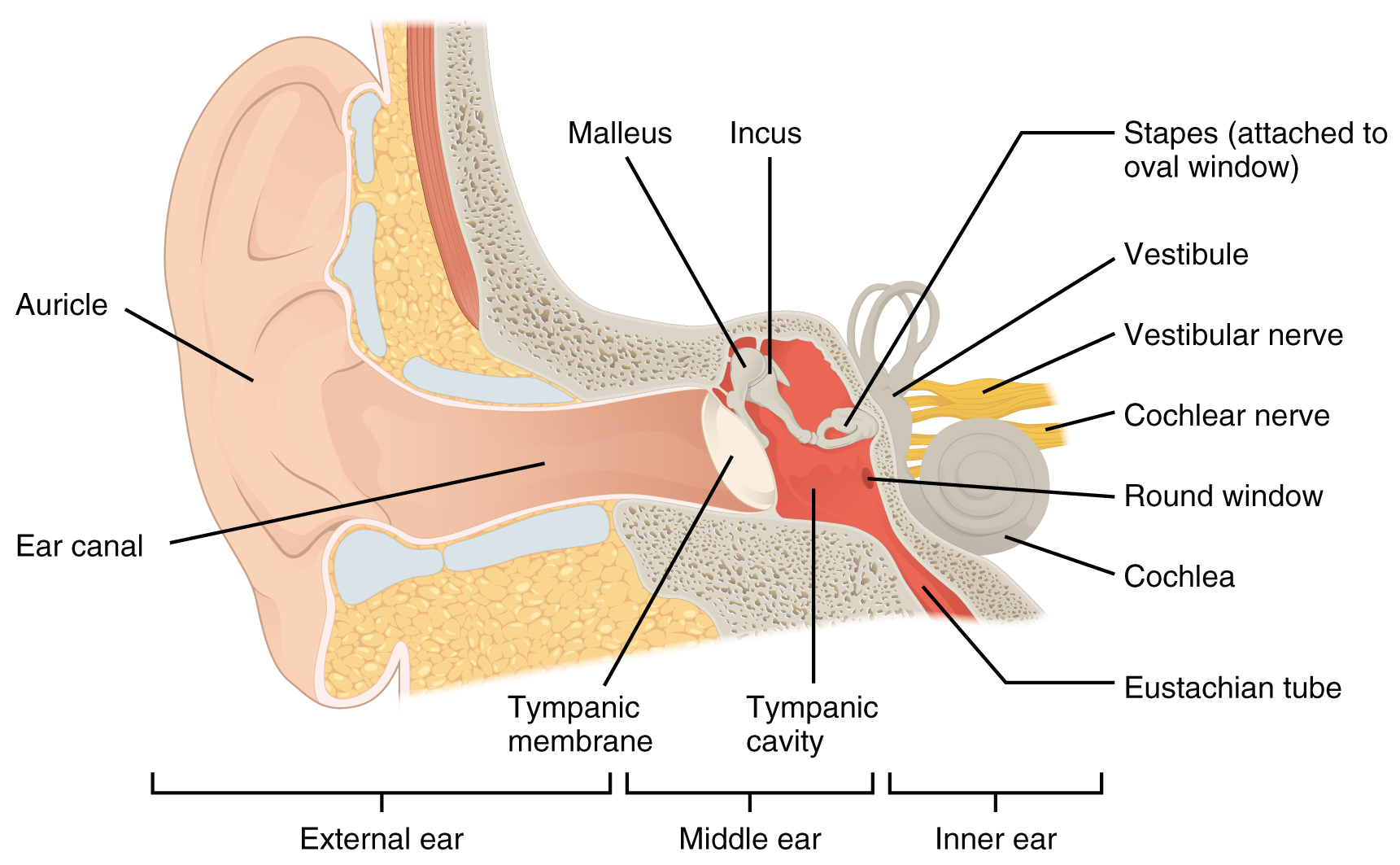
The inner ear is often described as a bony labyrinth, as it is composed of a series of canals embedded within the temporal bone. It has two separate regions, the cochlea and the vestibule, which are responsible for hearing and balance, respectively. The neural signals from these two regions are relayed to the brain stem through separate fiber bundles. However, these two distinct bundles travel together from the inner ear to the brain stem as the vestibulocochlear nerve. Sound is transduced into neural signals within the cochlear region of the inner ear, which contains the sensory neurons of the spiral ganglia. These ganglia are located within the spiral-shaped cochlea of the inner ear. The cochlea is attached to the stapes through the oval window.
The oval window is located at the beginning of a fluid-filled tube within the cochlea called the scala vestibuli. The scala vestibuli extends from the oval window, travelling above the cochlear duct, which is the central cavity of the cochlea that contains the sound-transducing neurons. At the uppermost tip of the cochlea, the scala vestibuli curves over the top of the cochlear duct. The fluid-filled tube, now called the scala tympani, returns to the base of the cochlea, this time travelling under the cochlear duct. The scala tympani ends at the round window, which is covered by a membrane that contains the fluid within the scala. As vibrations of the ossicles travel through the oval window, the fluid of the scala vestibuli and scala tympani moves in a wave-like motion. The frequency of the fluid waves match the frequencies of the sound waves (Figure 15.3.2). The membrane covering the round window will bulge out or pucker in with the movement of the fluid within the scala tympani.
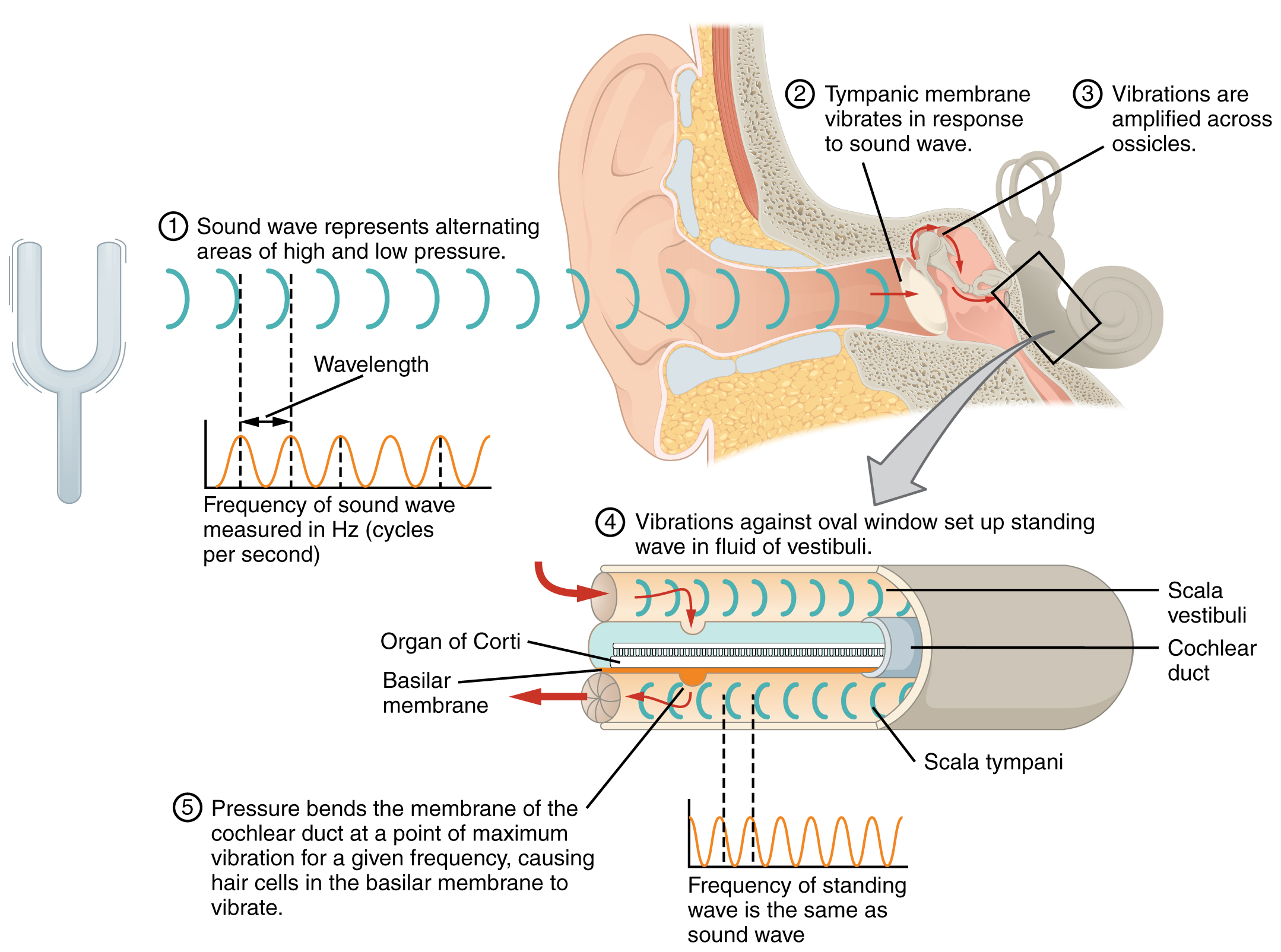
A cross-sectional view of the cochlea shows that the scala vestibuli and scala tympani run along both sides of the cochlear duct (Figure 15.3.3). The cochlear duct contains several organs of Corti, which tranduce the wave motion of the two scala into neural signals. The organs of Corti lie on top of the basilar membrane, which is the side of the cochlear duct located between the organs of Corti and the scala tympani. As the fluid waves move through the scala vestibuli and scala tympani, the basilar membrane moves at a specific spot, depending on the frequency of the waves. Higher frequency waves move the region of the basilar membrane that is close to the base of the cochlea. Lower frequency waves move the region of the basilar membrane that is near the tip of the cochlea.
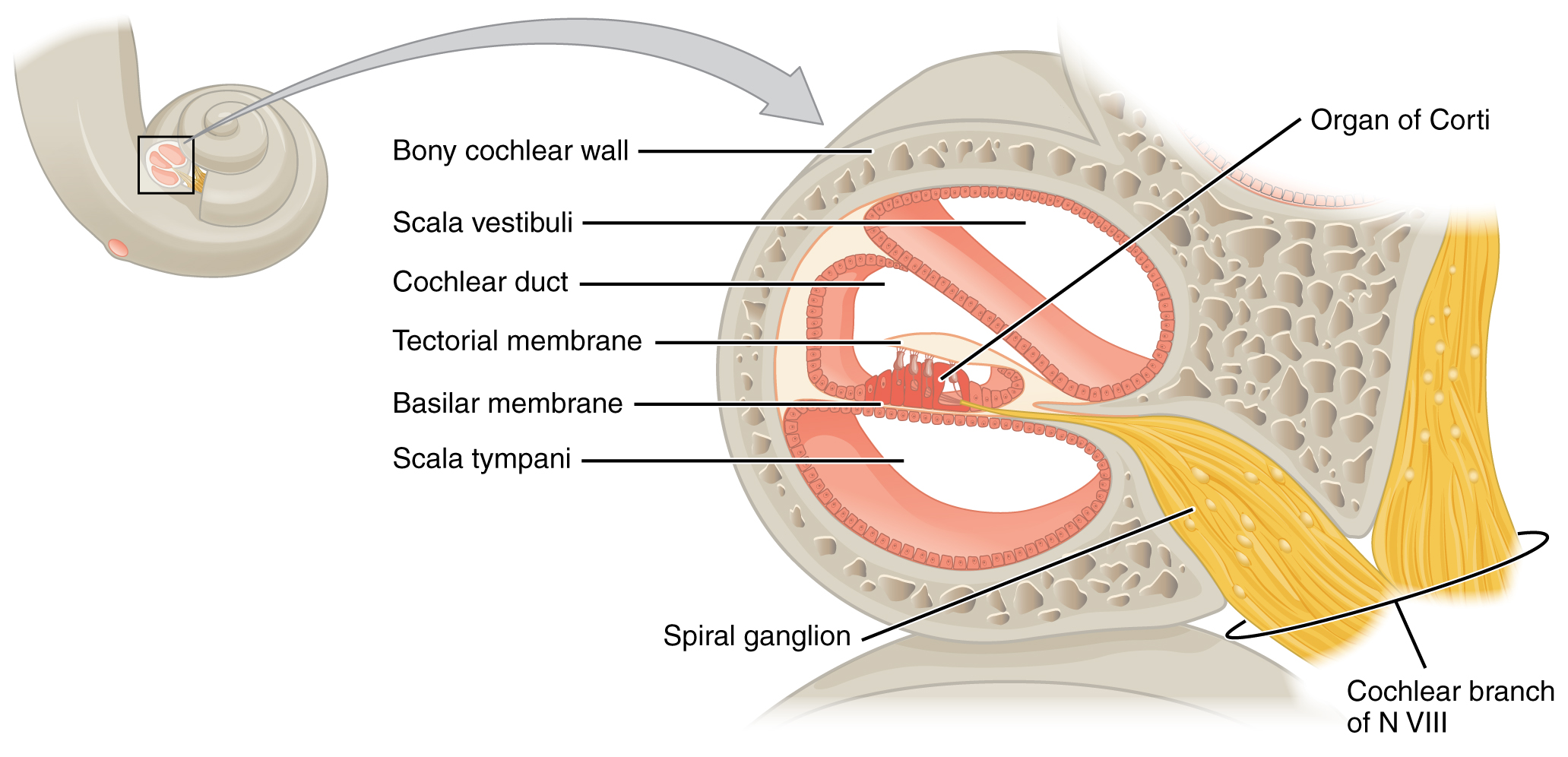
The organs of Corti contain hair cells, which are named for the hair-like stereocilia extending from the cell’s apical surfaces (Figure 15.3.4). The stereocilia are an array of microvilli-like structures arranged from tallest to shortest. Protein fibers tether adjacent hairs together within each array, such that the array will bend in response to movements of the basilar membrane. The stereocilia extend up from the hair cells to the overlying tectorial membrane, which is attached medially to the organ of Corti. When the pressure waves from the scala move the basilar membrane, the tectorial membrane slides across the stereocilia. This bends the stereocilia either toward or away from the tallest member of each array. When the stereocilia bend toward the tallest member of their array, tension in the protein tethers opens ion channels in the hair cell membrane. This will depolarize the hair cell membrane, triggering nerve impulses that travel down the afferent nerve fibers attached to the hair cells. When the stereocilia bend toward the shortest member of their array, the tension on the tethers slackens and the ion channels close. When no sound is present, and the stereocilia are standing straight, a small amount of tension still exists on the tethers, keeping the membrane potential of the hair cell slightly depolarized.
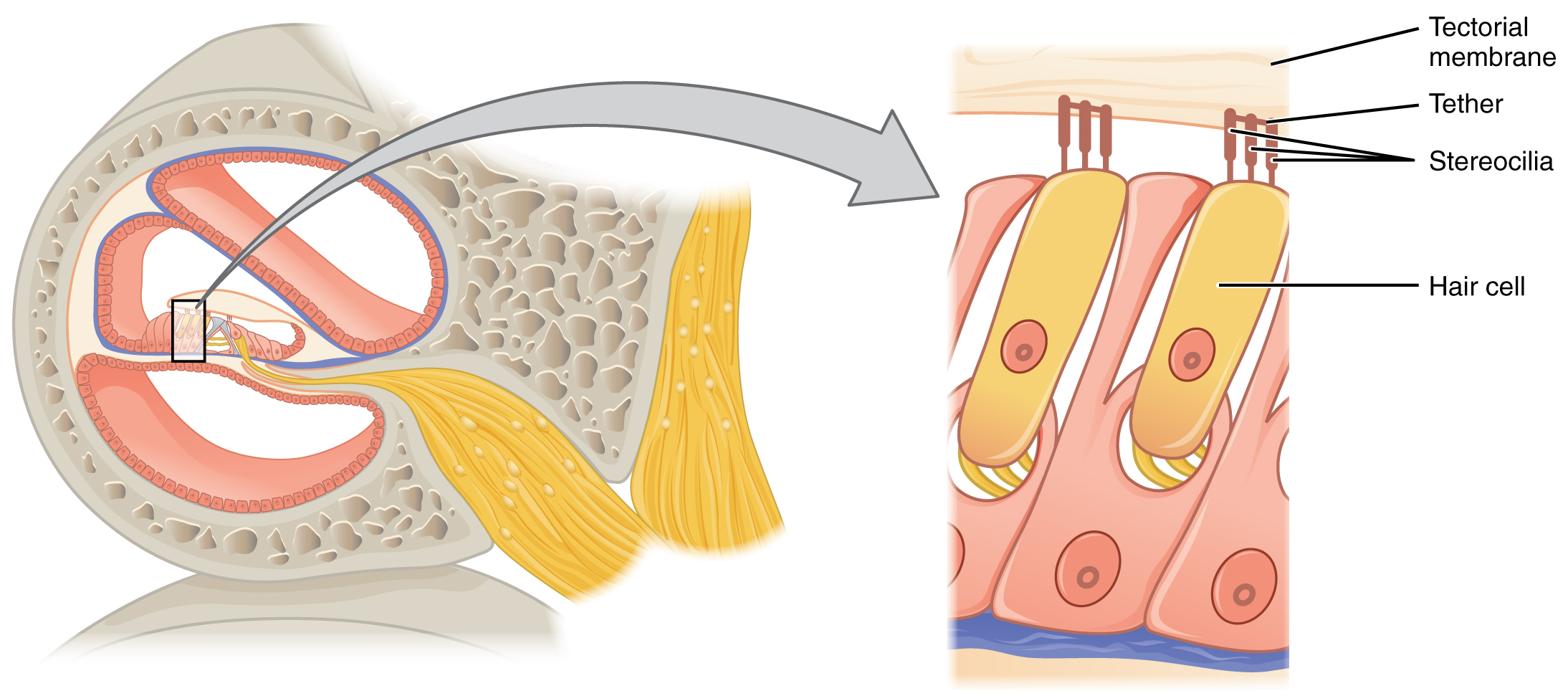
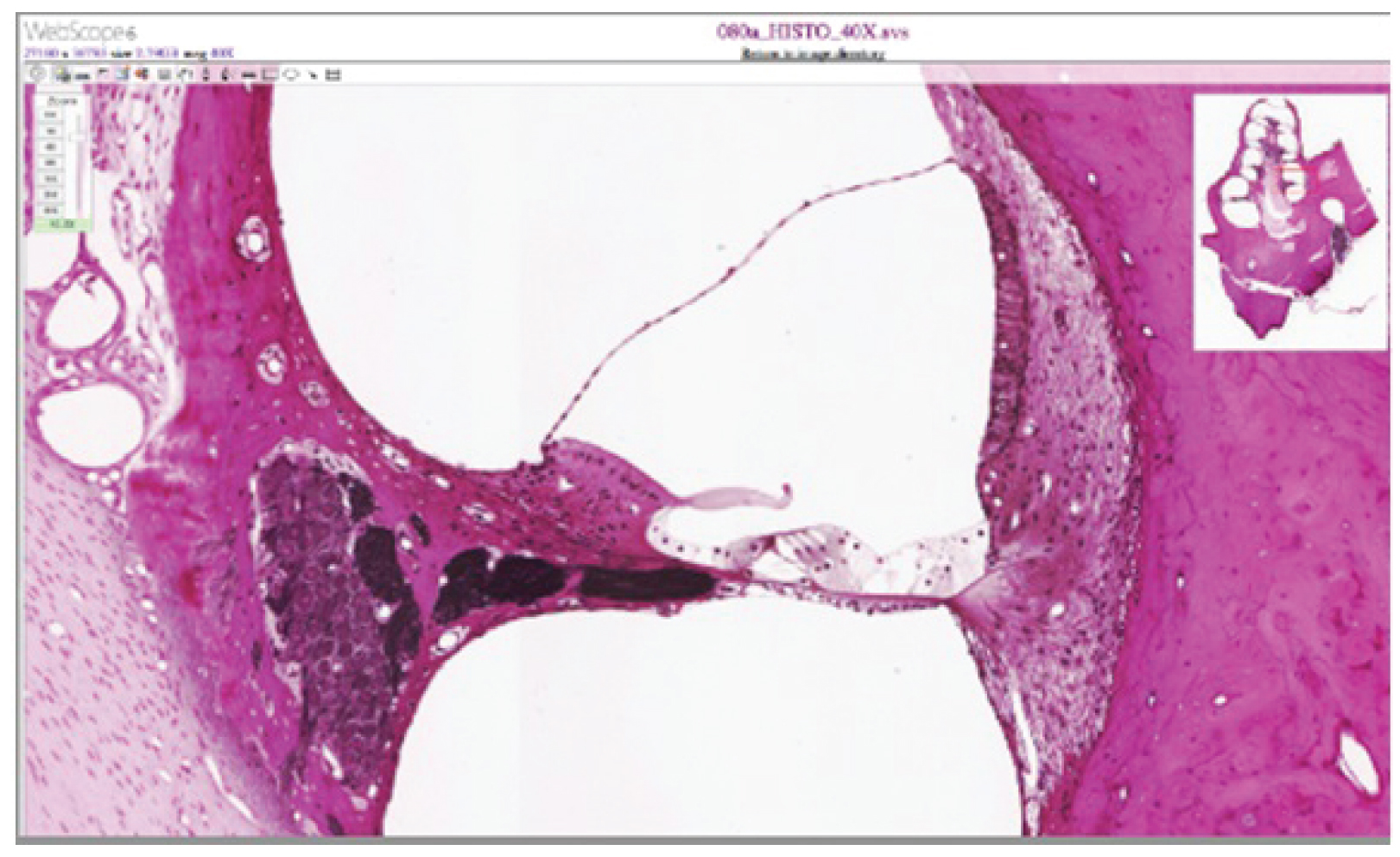
External Website
View the University of Michigan WebScope at http://virtualslides.med.umich.edu/Histology/Central%20Nervous%20System/080a_HISTO_40X.svs/view.apml to explore the tissue sample in greater detail. The basilar membrane is the thin membrane that extends from the central core of the cochlea to the edge. What is anchored to this membrane so that they can be activated by movement of the fluids within the cochlea?
As stated above, a given region of the basilar membrane will only move if the incoming sound is at a specific frequency. Because the tectorial membrane only moves where the basilar membrane moves, the hair cells in this region will also only respond to sounds of this specific frequency. Therefore, as the frequency of a sound changes, different hair cells are activated all along the basilar membrane. The cochlea encodes auditory stimuli for frequencies between 20 and 20,000 Hz, which is the range of sound that human ears can detect. The unit of Hertz measures the frequency of sound waves in terms of cycles produced per second. Frequencies as low as 20 Hz are detected by hair cells at the apex, or tip, of the cochlea. Frequencies in the higher ranges of 20 KHz are encoded by hair cells at the base of the cochlea, close to the round and oval windows (Figure 15.3.6). Most auditory stimuli contain a mixture of sounds at a variety of frequencies and intensities (represented by the amplitude of the sound wave). The hair cells along the length of the cochlear duct, which are each sensitive to a particular frequency, allow the cochlea to separate auditory stimuli by frequency, just as a prism separates visible light into its component colors.
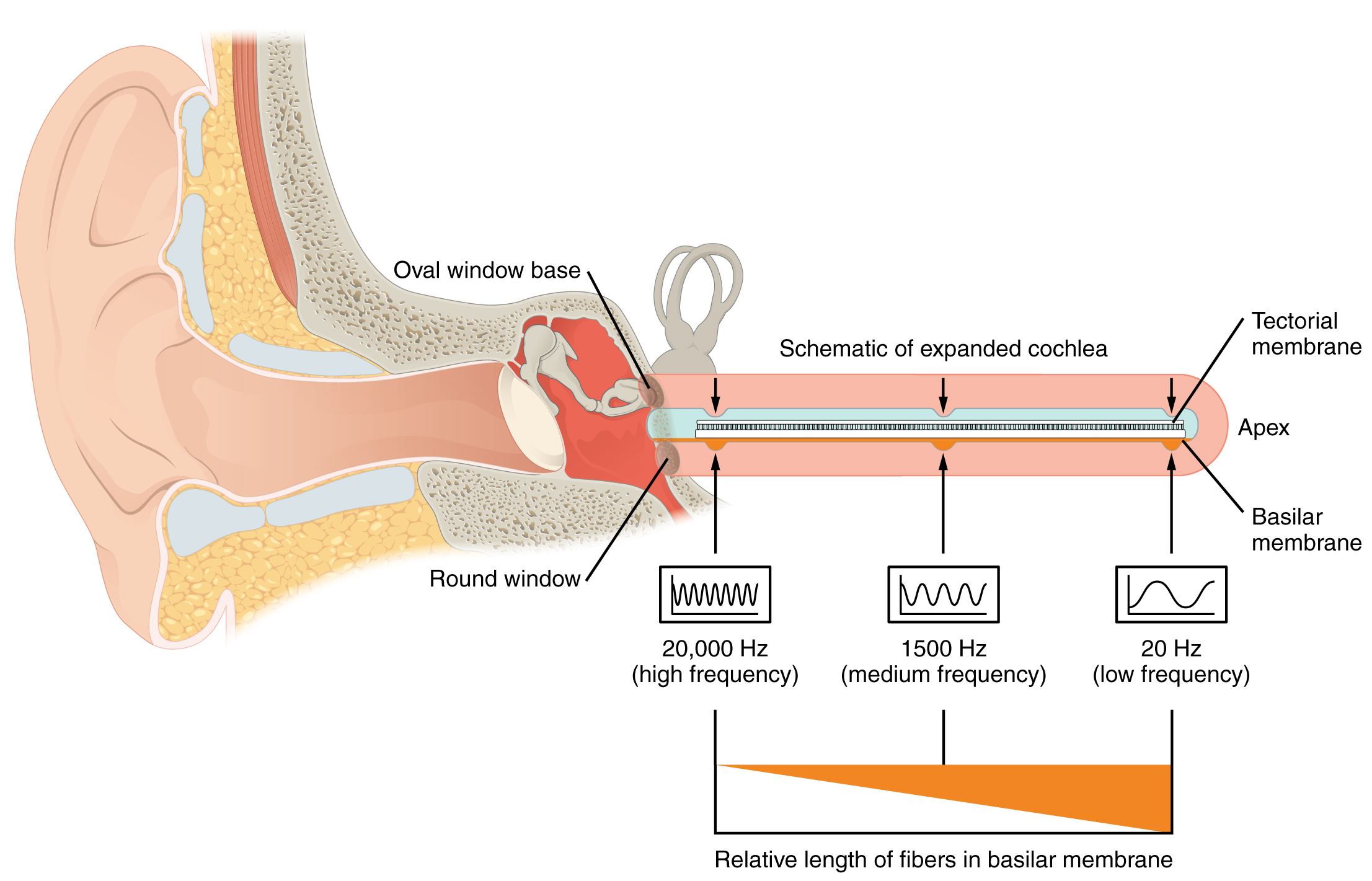
External Website
Watch this video to learn more about how the structures of the ear convert sound waves into a neural signal by moving the “hairs,” or stereocilia, of the cochlear duct. Specific locations along the length of the duct encode specific frequencies, or pitches. The brain interprets the meaning of the sounds we hear as music, speech, noise, etc. Which ear structures are responsible for the amplification and transfer of sound from the external ear to the inner ear?
External Website
Watch this animation to learn more about the inner ear and to see the cochlea unroll, with the base at the back of the image and the apex at the front. Specific wavelengths of sound cause specific regions of the basilar membrane to vibrate, much like the keys of a piano produce sound at different frequencies. Based on the animation, where do frequencies—from high to low pitches—cause activity in the hair cells within the cochlear duct?
Central Processing of Hearing Information
The sensory pathway for audition travels along the vestibulocochlear nerve, which synapses with neurons in the cochlear nuclei of the superior medulla. Within the brain stem, input from either ear is combined to extract location information from the auditory stimuli. Whereas the initial auditory stimuli received at the cochlea strictly represent the frequency—or pitch—of the stimuli, the locations of sounds can be determined by comparing information arriving at both ears.
Sound localization is a feature of central processing in the auditory nuclei of the brain stem. Sound localization is achieved by the brain calculating the interaural time difference and the interaural intensity difference. A sound originating from a specific location will arrive at each ear at different times, unless the sound is directly in front of the listener. If the sound source is slightly to the left of the listener, the sound will arrive at the left ear microseconds before it arrives at the right ear (Figure 15.3.7). This time difference is an example of an interaural time difference. Also, the sound will be slightly louder in the left ear than in the right ear because some of the sound waves reaching the opposite ear are blocked by the head. This is an example of an interaural intensity difference.
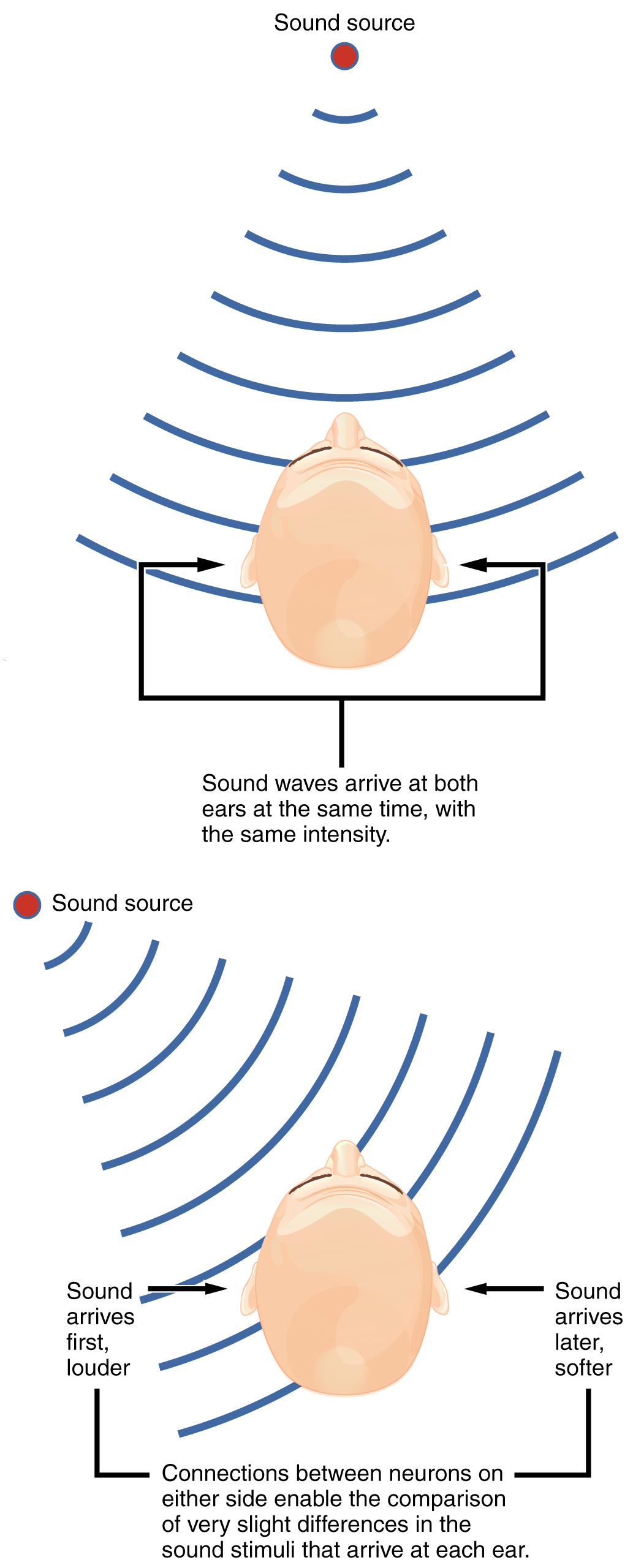
Auditory processing continues on to a nucleus in the midbrain called the inferior colliculus. Axons from the inferior colliculus project to two locations, the thalamus and the superior colliculus. The medial geniculate nucleus of the thalamus receives the auditory information and then projects that information to the auditory cortex in the temporal lobe of the cerebral cortex. The superior colliculus receives input from the visual and somatosensory systems, as well as the ears, to initiate stimulation of the muscles that turn the head and neck toward the auditory stimulus.
Review Questions
This work, Anatomy & Physiology, is adapted from Anatomy & Physiology by OpenStax, licensed under CC BY. This edition, with revised content and artwork, is licensed under CC BY-SA except where otherwise noted.
Images, from Anatomy & Physiology by OpenStax, are licensed under CC BY except where otherwise noted.
Access the original for free at https://openstax.org/books/anatomy-and-physiology/pages/1-introduction.