19.3 Bacterial Infections of the Gastrointestinal Tract
Learning Objectives
- Identify the most common bacteria that can cause infections of the GI tract
- Compare the major characteristics of specific bacterial diseases affecting the GI tract
A wide range of gastrointestinal diseases are caused by bacterial contamination of food. Recall that foodborne disease can arise from either infection or intoxication. In both cases, bacterial toxins are typically responsible for producing disease signs and symptoms. The distinction lies in where the toxins are produced. In an infection, the microbial agent is ingested, colonizes the gut, and then produces toxins that damage host cells. In an intoxication, bacteria produce toxins in the food before it is ingested. In either case, the toxins cause damage to the cells lining the gastrointestinal tract, typically the colon. This leads to the common signs and symptoms of diarrhea or watery stool and abdominal cramps, or the more severe dysentery. Symptoms of foodborne diseases also often include nausea and vomiting, which are mechanisms the body uses to expel the toxic materials.
Most bacterial gastrointestinal illness is short-lived and self-limiting; however, loss of fluids due to severe diarrheal illness can lead to dehydration that can, in some cases, be fatal without proper treatment. Oral rehydration therapy with electrolyte solutions is an essential aspect of treatment for most patients with GI disease, especially in children and infants.
Staphylococcal Food Poisoning
Staphylococcal food poisoning is one form of food intoxication. When Staphylococcus aureus grows in food, it may produce enterotoxins that, when ingested, can cause symptoms such as nausea, diarrhea, cramping, and vomiting within one to six hours. In some severe cases, it may cause headache, dehydration, and changes in blood pressure and heart rate. Signs and symptoms resolve within 24 to 48 hours. S. aureus is often associated with a variety of raw or undercooked and cooked foods including meat (e.g., canned meat, ham, and sausages) and dairy products (e.g., cheeses, milk, and butter). It is also commonly found on hands and can be transmitted to prepared foods through poor hygiene, including poor handwashing and the use of contaminated food preparation surfaces, such as cutting boards. The greatest risk is for food left at a temperature below 60 °C (140 °F), which allows the bacteria to grow. Cooked foods should generally be reheated to at least 60 °C (140 °F) for safety and most raw meats should be cooked to even higher internal temperatures (Figure 19.10).
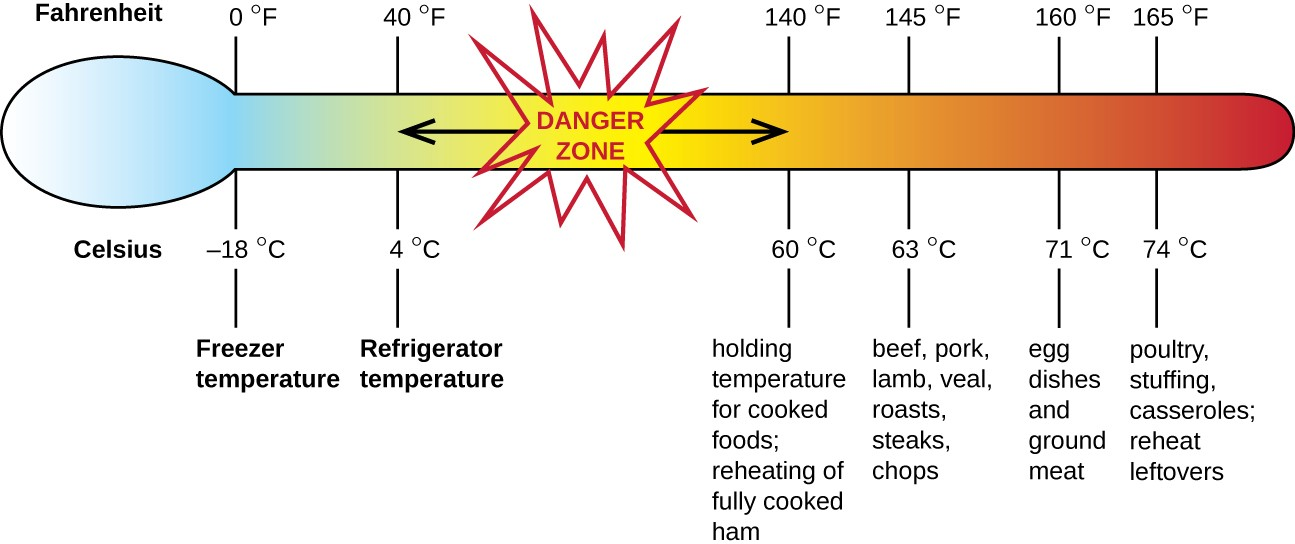
There are at least 21 Staphylococcal enterotoxins and Staphylococcal enterotoxin-like toxins that can cause food intoxication. The enterotoxins are proteins that are resistant to low pH, allowing them to pass through the stomach. They are heat stable and are not destroyed by boiling at 100 °C. Even though the bacterium itself may be killed, the enterotoxins alone can cause vomiting and diarrhea, although the mechanisms are not fully understood. At least some of the symptoms may be caused by the enterotoxin functioning as a superantigen and provoking a strong immune response by activating T cell proliferation.
The rapid onset of signs and symptoms helps to diagnose this foodborne illness. Because the bacterium does not need to be present for the toxin to cause symptoms, diagnosis is confirmed by identifying the toxin in a food sample or in biological specimens (feces or vomitus) from the patient. Serological techniques, including ELISA, can also be used to identify the toxin in food samples.
The condition generally resolves relatively quickly, within 24 hours, without treatment. In some cases, supportive treatment in a hospital may be needed.
- How can S. aureus cause food intoxication?
E. coli Infections
The gram-negative rod Escherichia coli is a common member of the normal microbiota of the colon. Although the vast majority of E. coli strains are helpful commensal bacteria, some can be pathogenic and may cause dangerous diarrheal disease. The pathogenic strains have additional virulence factors such as type 1 fimbriae that promote colonization of the colon or may produce toxins. These virulence factors are acquired through horizontal gene transfer.
Extraintestinal disease can result if the bacteria spread from the gastrointestinal tract. Although these bacteria can be spread from person to person, they are often acquired through contaminated food or water. There are six recognized pathogenic groups of E. coli, but we will focus here on the four that are most commonly transmitted through food and water.
Enterotoxigenic E. coli (ETEC), also known as traveler’s diarrhea, causes diarrheal illness and is common in less developed countries. In Mexico, ETEC infection is called Montezuma’s Revenge. Following ingestion of contaminated food or water, infected individuals develop a watery diarrhea, abdominal cramps, malaise (a feeling of being unwell), and a low fever. ETEC produces a heat-stable enterotoxin similar to cholera toxin, and adhesins called colonization factors that help the bacteria to attach to the intestinal wall. Some strains of ETEC also produce heat-labile toxins. The disease is usually relatively mild and self-limiting. Diagnosis involves culturing and PCR. If needed, antibiotic treatment with fluoroquinolones, doxycycline, rifaximin, and trimethoprim-sulfamethoxazole (TMP/SMZ) may shorten infection duration. However, antibiotic resistance is a problem.
Enteroinvasive E. coli (EIEC) is very similar to shigellosis, including its pathogenesis of intracellular invasion into intestinal epithelial tissue. This bacterium carries a large plasmid that is involved in epithelial cell penetration. The illness is usually self-limiting, with symptoms including watery diarrhea, chills, cramps, malaise, fever, and dysentery. Culturing and PCR testing can be used for diagnosis. Antibiotic treatment is not recommended, so supportive therapy is used if needed.
Enteropathogenic E. coli (EPEC) can cause a potentially fatal diarrhea, especially in infants and those in less developed countries. Fever, vomiting, and diarrhea can lead to severe dehydration. These E. coli inject a protein that attaches to the surface of the intestinal epithelial cells and triggers rearrangement of host cell actin from microvilli to pedestals. The protein also happens to be the receptor for a surface protein produced by EPEC, thereby allowing E. coli to “sit” on the pedestal. As with ETEC, diagnosis involves culturing and PCR. Treatment is similar to that for ETEC.
The most dangerous strains are enterohemorrhagic E. coli (EHEC), which are the strains capable of causing epidemics. In particular, the strain O157:H7 has been responsible for several recent outbreaks. Recall that the O and H refer to surface antigens that contribute to pathogenicity and trigger a host immune response (“O” refers to the O-side chain of the lipopolysaccharide and the “H” refers to the flagella). Similar to EPEC, EHEC also forms pedestals. EHEC also produces a Shiga-like toxin. Because the genome of this bacterium has been sequenced, it is known that the Shiga toxin genes were most likely acquired through transduction (horizontal gene transfer). The Shiga toxin genes originated from Shigella dysenteriae. Prophage from a bacteriophage that previously infected Shigella integrated into the chromosome of E. coli. The Shiga-like toxin is often called verotoxin.
EHEC can cause disease ranging from relatively mild to life-threatening. Symptoms include bloody diarrhea with severe cramping, but no fever. Although it is often self-limiting, it can lead to hemorrhagic colitis and profuse bleeding. One possible complication is HUS. Diagnosis involves culture, often using MacConkey with sorbitol agar to differentiate between E. coli O157:H7, which does not ferment sorbitol, and other less virulent strains of E. coli that can ferment sorbitol.
Antibiotic therapy is not recommended and may worsen HUS because of the toxins released when the bacteria are killed, so supportive therapies must be used. Table 19.1 summarizes the characteristics of the four most common pathogenic groups.
Group |
Virulence Factors and Genes |
Signs and Symptoms |
Diagnostic Tests |
Treatment |
---|---|---|---|---|
Enterotoxigenic E. coli (ETEC) |
Heat stable enterotoxin similar to cholera toxin |
Relatively mild, watery diarrhea |
Culturing, PCR |
Self-limiting; if needed, fluoroquinolones, doxycycline, rifaximin, TMP/SMZ; antibiotic resistance is a problem |
Enteroinvasive E. coli (EIEC) |
Inv (invasive plasmid) genes |
Relatively mild, watery diarrhea; dysentery or inflammatory colitis may occur |
Culturing, PCR; testing for inv gene; additional assays to distinguish from Shigella |
Supportive therapy only; antibiotics not recommended |
Enteropathogenic E. coli (EPEC) |
Locus of enterocyte effacement (LEE) pathogenicity island |
Severe fever,vomiting, nonbloody diarrhea, dehydration; potentially fatal |
Culturing, PCR; detection of LEE lacking Shiga-like toxingenes |
Self-limiting; if needed, fluoroquinolones, doxycycline, rifaximin (TMP/SMZ); antibiotic resistance is a problem |
Enterohemorrhagic E. coli (EHEC) |
Verotoxin |
May be mild or very severe; bloody diarrhea; may result in HUS |
Culturing; plate onMacConkey agar with sorbitol agar as it does not ferment sorbitol; PCR detection of LEE containing Shiga-like toxin genes |
Antibiotics are not recommended due to the risk of HUS |
- Compare and contrast the four main E. coli groups associated with illness.
Peptic Ulcers
The gram-negative bacterium Helicobacter pylori is able to tolerate the acidic environment of the human stomach and has been shown to be a major cause of peptic ulcers, which are ulcers of the stomach or duodenum. The bacterium is also associated with increased risk of stomach cancer (Figure 19.11). According to the CDC, approximately two- thirds of the population is infected with H. pylori, but less than 20% have a risk of developing ulcers or stomach cancer. H. pylori is found in approximately 80% of stomach ulcers and in over 90% of duodenal ulcers.[1]
H. pylori colonizes epithelial cells in the stomach using pili for adhesion. These bacteria produce urease, which stimulates an immune response and creates ammonia that neutralizes stomach acids to provide a more hospitable microenvironment. The infection damages the cells of the stomach lining, including those that normally produce the protective mucus that serves as a barrier between the tissue and stomach acid. As a result, inflammation (gastritis) occurs and ulcers may slowly develop. Ulcer formation can also be caused by toxin activity. It has been reported that 50% of clinical isolates of H. pylori have detectable levels of exotoxin activity in vitro.[2]
Signs and symptoms include nausea, lack of appetite, bloating, burping, and weight loss. Bleeding ulcers may produce dark stools. If no treatment is provided, the ulcers can become deeper, more tissues can be involved, and stomach perforation can occur. Because perforation allows digestive enzymes and acid to leak into the body, it is a very serious condition.
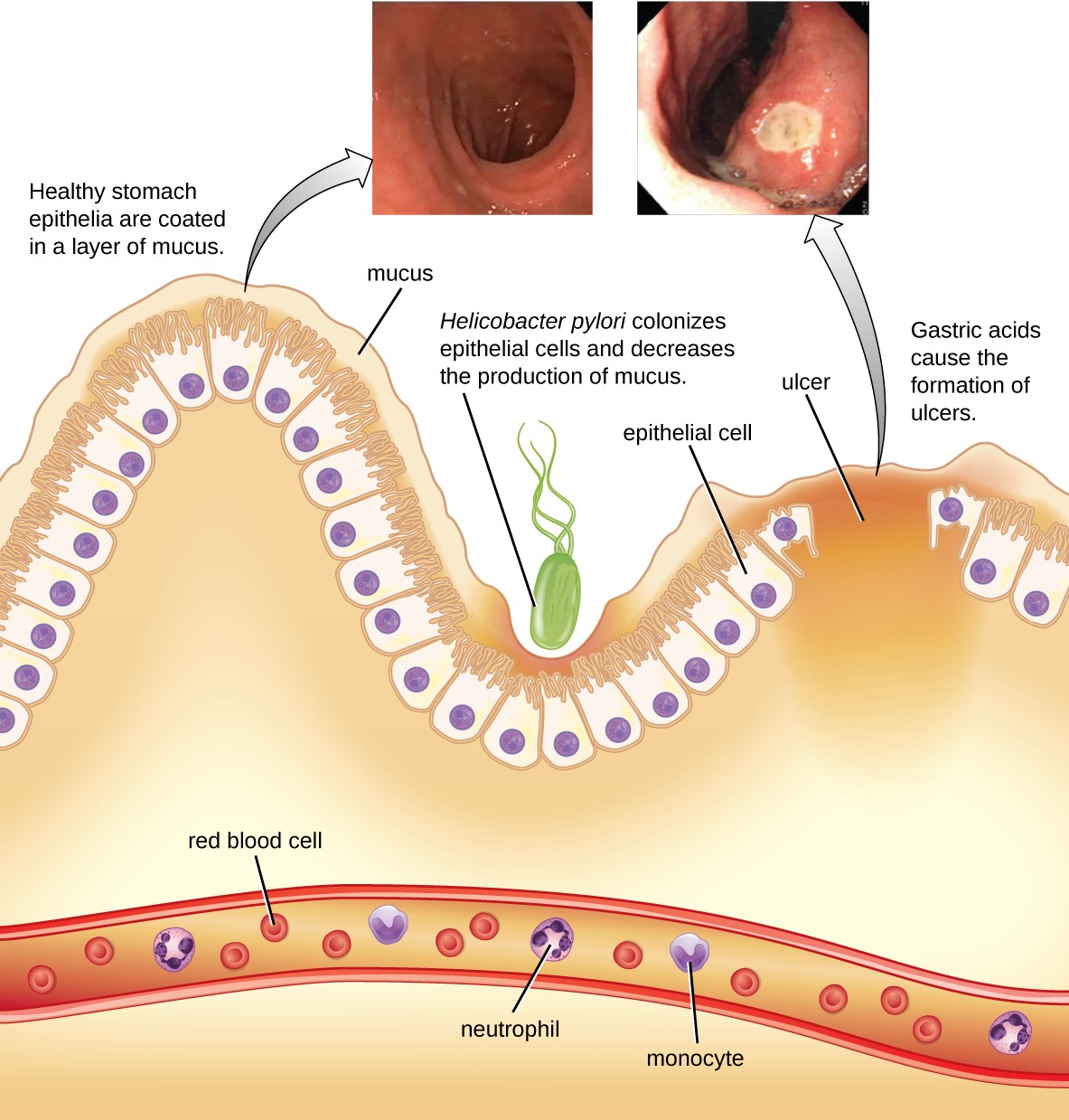
To diagnose H. pylori infection, multiple methods are available. In a breath test, the patient swallows radiolabeled urea. If H. pylori is present, the bacteria will produce urease to break down the urea. This reaction produces radiolabeled carbon dioxide that can be detected in the patient’s breath. Blood testing can also be used to detect antibodies to H. pylori. The bacteria themselves can be detected using either a stool test or a stomach wall biopsy.
Antibiotics can be used to treat the infection. However, unique to H. pylori, the recommendation from the US Food and Drug Administration is to use a triple therapy. The current protocols are 10 days of treatment with omeprazole, amoxicillin, and clarithromycin (OAC); 14 days of treatment with bismuth subsalicylate, metronidazole, and tetracycline (BMT); or 10 or 14 days of treatment with lansoprazole, amoxicillin, and clarithromycin (LAC). Omeprazole, bismuth subsalicylate, and lansoprazole are not antibiotics but are instead used to decrease acid levels because H. pylori prefers acidic environments.
Although treatment is often valuable, there are also risks to H. pylori eradication. Infection with H. pylori may actually protect against some cancers, such as esophageal adenocarcinoma and gastroesophageal reflux disease.[3][4]
- How does H. pylori cause peptic ulcers?
Clostridium difficile
Clostridium difficile is a gram-positive rod that can be a commensal bacterium as part of the normal microbiota of healthy individuals. When the normal microbiota is disrupted by long-term antibiotic use, it can allow the overgrowth of this bacterium, resulting in antibiotic-associated diarrhea caused by C. difficile. Antibiotic-associated diarrhea can also be considered a nosocomial disease. Patients at the greatest risk of C. difficile infection are those who are immunocompromised, have been in health-care settings for extended periods, are older, have recently taken antibiotics, have had gastrointestinal procedures done, or use proton pump inhibitors, which reduce stomach acidity and allow proliferation of C. difficile. Because this species can form endospores, it can survive for extended periods of time in the environment under harsh conditions and is a considerable concern in health-care settings.
This bacterium produces two toxins, Clostridium difficile toxin A (TcdA) and Clostridium difficile toxin B (TcdB). These toxins inactivate small GTP-binding proteins, resulting in actin condensation and cell rounding, followed by cell death. Infections begin with focal necrosis, then ulceration with exudate, and can progress to pseudomembranous colitis, which involves inflammation of the colon and the development of a pseudomembrane of fibrin containing dead epithelial cells and leukocytes (Figure 19.12). Watery diarrhea, dehydration, fever, loss of appetite, and abdominal pain can result. Perforation of the colon can occur, leading to septicemia, shock, and death. C. difficile is also associated with necrotizing enterocolitis in premature babies and neutropenic enterocolitis associated with cancer therapies.
Diagnosis is made by considering the patient history (such as exposure to antibiotics), clinical presentation, imaging, endoscopy, lab tests, and other available data. Detecting the toxin in stool samples is used to confirm diagnosis. Although culture is preferred, it is rarely practical in clinical practice because the bacterium is an obligate anaerobe. Nucleic acid amplification tests, including PCR, are considered preferable to ELISA testing for molecular analysis.
The first step of conventional treatment is to stop antibiotic use, and then to provide supportive therapy with electrolyte replacement and fluids. Metronidazole is the preferred treatment if the C. difficile diagnosis has been confirmed. Vancomycin can also be used, but it should be reserved for patients for whom metronidazole was ineffective or who meet other criteria (e.g., under 10 years of age, pregnant, or allergic to metronidazole).
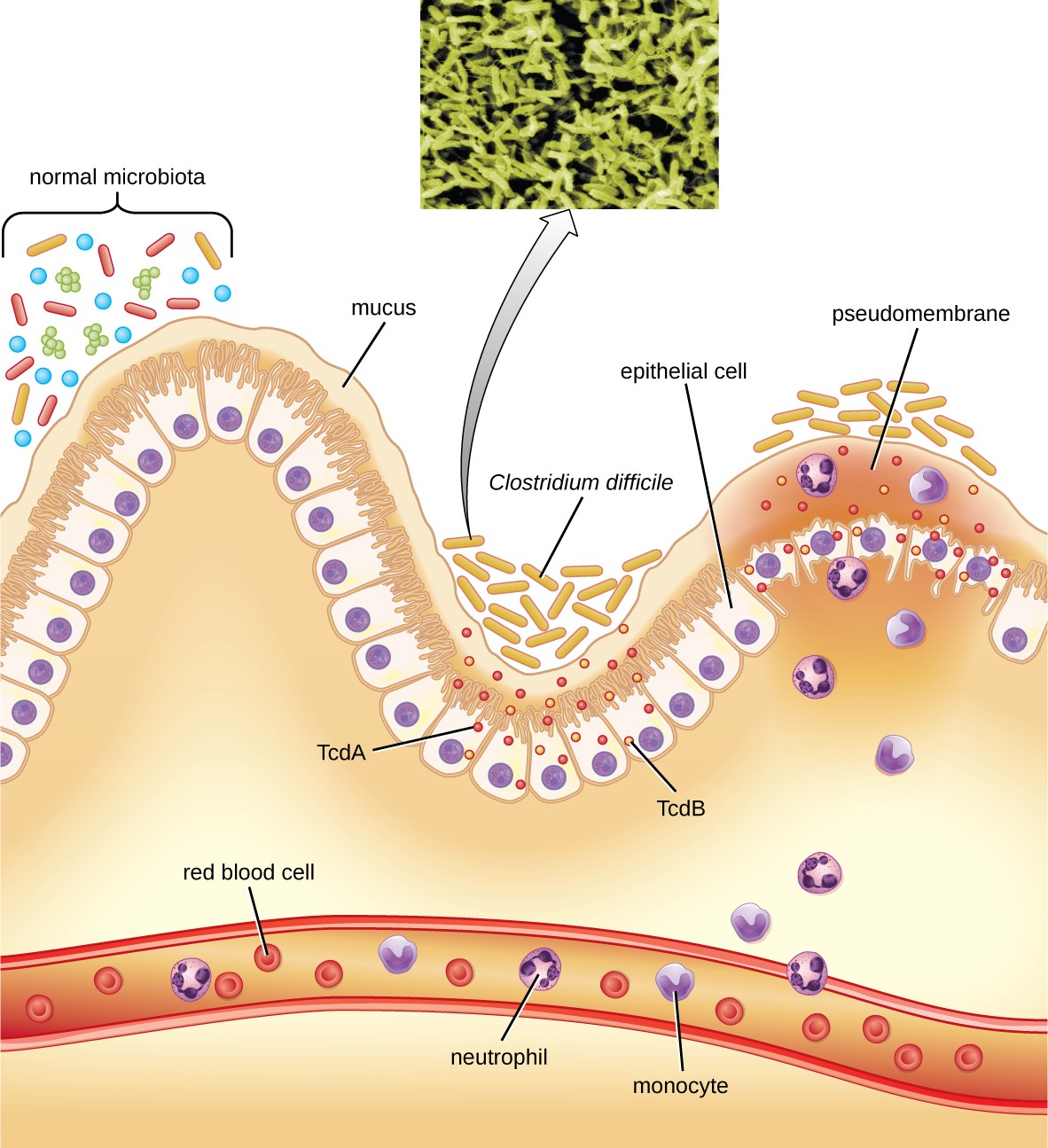
A newer approach to treatment, known as a fecal transplant, focuses on restoring the microbiota of the gut in order to combat the infection. In this procedure, a healthy individual donates a stool sample, which is mixed with saline and transplanted to the recipient via colonoscopy, endoscopy, sigmoidoscopy, or enema. It has been reported that this procedure has greater than 90% success in resolving C. difficile infections.[5]
- How does antibiotic use lead to C. difficile infections?
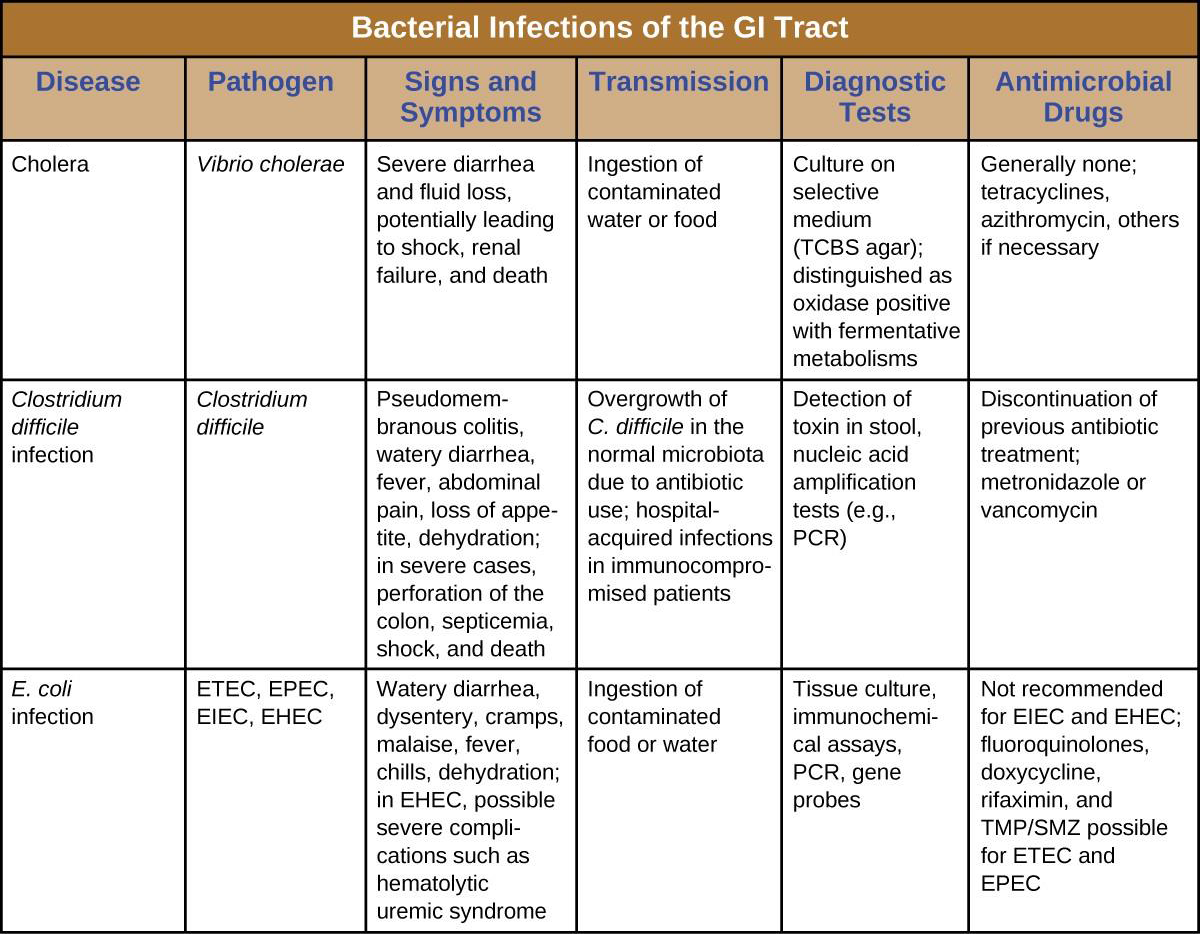
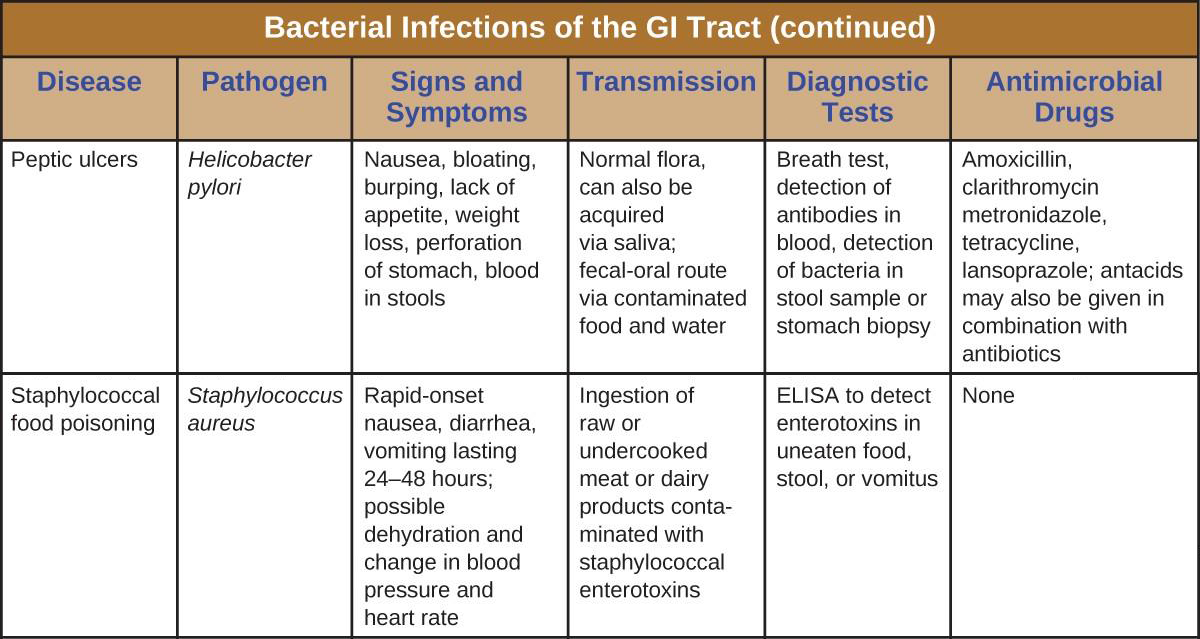
- Centers for Disease Control and Prevention. “Helicobacter pylori: Fact Sheet for Health Care Providers.” Updated July 1998. http://www.cdc.gov/ulcer/files/hpfacts.pdf. ↵
- T. L. Cover. “The Vacuolating Cytotoxin of Helicobacter pylori.” Molecular Microbiology 20 (1996) 2: pp. 241–246. http://www.ncbi.nlm.nih.gov/pubmed/8733223. ↵
- Martin J. Blaser. “Disappearing Microbiota: Helicobacter pylori Protection against Esophageal Adenocarcinoma.” Cancer Prevention Research 1 (2008) 5: pp. 308–311. http://cancerpreventionresearch.aacrjournals.org/content/1/5/308.full.pdf+html. ↵
- Ivan F. N. Hung and Benjamin C. Y. Wong. “Assessing the Risks and Benefits of Treating Helicobacter pylori Infection.” Therapeutic Advances in Gastroenterology 2 (2009) 3: pp, 141–147. doi: 10.1177/1756283X08100279. ↵
- Faith Rohlke and Neil Stollman. “Fecal Microbiota Transplantation in Relapsing Clostridium difficile Infection,” Therapeutic Advances in Gastroenterology 5 (2012) 6: 403–420. doi: 10.1177/1756283X12453637. ↵