8 Field Techniques for Population Sampling and Estimation
Field techniques refer to the standardized methods employed to select, count, measure, capture, mark, and observe individuals sampled from the target population for the purpose of collecting data required to achieve study objectives. The term also includes methods used to collect voucher specimens, tissue samples, and habitat data. The choice of field techniques to use for a particular species or population is influenced by five major factors:
- Data needed to achieve inventory and monitoring objectives
- Spatial extent and duration of the project
- Life history and population characteristics
- Terrain and vegetation in the study area
- Budget constraints
Data Requirements
The types of data required to achieve inventory or monitoring objectives should be the primary consideration in selecting field techniques. Four categories of data collection are discussed below along with some suggestions for electing appropriate field techniques for each.
Occurrence and distribution data
For some population studies, simply determining whether a species is present in an area is sufficient for conducting the planned data analysis. For example, biologists attempting to conserve a threatened salamander may need to monitor the extent of the species’ range and degree of population fragmentation on a land ownership. One hypothetical approach is to map all streams in which the salamander is known to be present, as well as additional streams that may qualify as the habitat type for the species in the region. To monitor changes in salamander distribution, data collection could consist of a survey along randomly selected reaches in each of the streams to determine if at least one individual (or some alternative characteristic such as egg mass) is present. Using only a list that includes the stream reach (i.e., the unique identifier), the survey year, and an occupancy indicator variable, a biologist could prepare a time series of maps displaying all of the streams by year and distinguish the subset of streams that were known to be occupied by the salamander. Such an approach could support a qualitative assessment of changes in the species distribution pattern, thereby attaining the program’ objectives, and generate new hypotheses as to the cause of the observed changes.
It is far easier to determine if there is at least one individual of the target species on a sampling unit than it is to count all of the individuals. Determining with confidence that a species is not present on a sampling unit also requires more intensive sampling than collecting count or frequency data because it is so difficult to dismiss the possibility that an individual eluded detection. Probability of occurrence can be estimated using approaches such as those described by MacKenzie and Royale (2005). MacKenzie (2005) offered an excellent overview for managers of the trade-off between number of units sampled per year and the number of years (or other unit of time) for which the study is to be conducted. The variation in the estimated trend in occupancy decreases as the number of years of data collection increases (Fig. 8.1). A similar level of precision can be achieved by surveying more units over fewer years vs. surveying fewer units over a longer period.
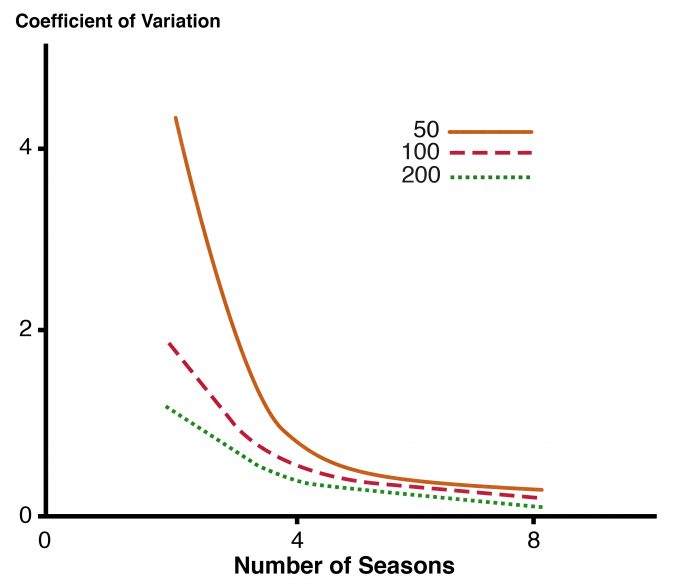
Population size and density
National policy on threatened and endangered species is ultimately directed toward efforts to increase or maintain the total number of individuals of the species within their natural geographic range (Suckling and Taylor 2006). Total population size and effective population size (i.e., the number of breeding individuals in a population; Lande and Barrowclough 1987) most directly indicate the degree of species endangerment and effectiveness of conservation policies and practices. Population size or more accurately density per unit area is usually used as the basis for trend analyses because changes in density integrate changes in natural mortality, exploitation, and habitat quality. In some circumstances, it may be feasible to conduct a census of all individuals of a particular species in an area to determine the population density. Typically however, population size and density parameters are estimated using statistical analyses based on only a sample of population members. Population densities of plants and sessile animals can be estimated from counts taken on plots or data describing the spacing between individuals (i.e. distance methods) and are relatively straightforward. Population analyses for many animal species must account for animal response to capture or observation, observer biases, and different detection probabilities among sub-populations. Pilot studies are usually required to collect the data necessary to address these factors in the analysis. Furthermore, mark-recapture studies, catch-per-unit effort surveys, and other estimation methods require multiple visits to sampling units (Pradel 1996). These considerations increase the complexity and cost of studies designed for population parameter estimation.
Abundance indices
The goals and objectives of some biological inventories and monitoring studies can be met with indices of population density or abundance, rather than population estimators. The difference between estimators and indices is that the former yield absolute values of population density while the latter provide relative measures of density that can be used to compare indices to populations among places or times. Indices are founded on the assumption that index values are closely associated with values of a population parameter, although the precise relationship between the index and parameter usually is not quantified. Examples of abundance or density indices are: plant canopy cover, numbers of individuals captured per 1000 trap nights, counts of individuals observed during a standardized unit of time, among many others. From a data collection perspective, density indices often require less sampling intensity and complexity than population estimation procedures. However, population indices are not comparable among different studies unless field techniques are strictly standardized. Furthermore, the assumption that an abundance index closely approximates population density is rarely tested (Seber 1982).
Fitness data
For rare or declining populations, estimates of survival in each life stage as well as reproductive rates are required. These data not only provide useful trigger points for estimating rates of decline (lambda) they also allow trigger points for removal of a species from a threatened or other legal status. Collecting these sorts of data is often labor intensive and expensive. In a study on northern spotted owls, for instance, millions of dollars have been spent collecting these types of data (Lint 2001). This is not particularly surprising as the types of data that would be necessary to understand the population dynamics of a bird are numerous and complicated to generate. Nest densities, clutch sizes, hatching rates, fledging rates, and survival rates to maturity and survival rates as reproductive adults would be a minimum data set. New approaches to estimating individual contributions to population growth and changes in distributions of quantitative traits and alleles include genetic analyses, which can lead to even more detailed understanding of the potential for a population to adapt to variations in environmental factors (Pelletier et al. 2009).
Research studies
Studies of habitat relationships or cause-and-effect responses require coordinated sampling of the target population and environmental measurements or stressors to which the population may respond. Data collection efforts tend to be complex, requiring multiple sampling protocols for the target population, study site attributes, and landscape pattern metrics. The funding required to conduct research studies typically limits their application to species or populations in greatest need of management planning such as those listed as threatened or endangered. Manipulative studies are often carried out to generate the necessary data, but when these focus on a threatened species, ethical questions regarding the conduct of the experiment placing the species at even great risk, at least locally, often emerge. Hence it is often monitoring of both environmental conditions and aspects of population density or fitness that are used to assess associations in trends between population parameters and environmental parameters.
Spatial Extent
Clearly the scope of inference will influence the type of sampling technique used. Breeding bird atlas techniques commonly use large grids placed over entire states to assess the occurrence of species in a grid cell. Such approaches and those of the Breeding Bird Survey (Sauer et al. 2008) can be conducted through volunteer efforts. On the other hand, monitoring the trends in reproductive rates of northern spotted owls, northern goshawks, or grizzly bears over their geographic ranges requires a huge budget to collect the level of population data over large areas needed to understand trends. Great care must be taken when deciding what technique to use because both budgets and sample size requirements enter into logistics. Indeed, it is often the tradeoff between more detailed data and the cost of producing those data that drive decisions regarding monitoring designs for species at risk.
Frequently Used Techniques for Sampling Animals
The array of techniques available to sample animals is vast and summarized elsewhere in techniques manuals (e.g., Bookhout 1994). We summarize a few examples of commonly used techniques, but strongly suggest that those of you developing monitoring plans do a more complete literature search on sampling of the species that are of most concern in your monitoring program. We first provide a brief overview of techniques used to sample vertebrates and then point out which techniques are commonly used among various taxonomic groups.
Aquatic Organisms
Some aquatic organisms can and have been monitored using techniques that are essentially identical to those used for terrestrial vertebrates. For instance, in Brazil, arapaima have been monitored using a point count technique that counts individuals as they surface for aerial breathing (Castello et al. 2009). Point counts were more logistically and economically feasible, were determined to more accurately represent population changes over time and led to more effective management, but a conventional mark-recapture technique was also attempted with the same fish species (Castello et al. 2009).
Yet cases such as the arapaima are uncommon because this species is detected when surfacing for aerial breathing, has a low enough population density in a small enough area to be counted effectively, and possesses certain subtle visual and acoustic characteristics that allow for the identification of individuals (Castello et al. 2009). Most techniques used to sample aquatic organisms are conceptually similar to those used to sample terrestrial organisms. But constraints placed on observers of dealing with sampling in or on the water and at various water depths require that many techniques be more specialized. There are a variety of techniques commonly used to sample fish and aquatic amphibians as well as aquatic invertebrates (Slack et al. 1973). A systematic assessment of stream reaches using either snorkel surveys (Hankin and Reeves 1988) or electrofishing equipment is commonly used in shallow streams and rivers (Cunjak et al. 1988).
In estuaries and large rivers, quantitative studies are often confounded by the high variability of fish populations and the high efficiency of fish sampling gear (Poizat and Baran 1997). In light of this, Poizat and Baran (1997) undertook a study assessing the efficacy of surveying fishermen compared with a scientist-managed gill-net sampling approach and determined that combining both approaches is the best way to increase confidence that observed trends are real. In other words, if both sets of survey data suggest the same trend, it is safer to infer that the trends are real than if the data sets suggest different trends or there exists but one type of data.
Manta tows, which are comparable to line-transect methods but must account for uniquely marine conditions such as turbidity of water, tides, and sea-condition characteristics, are often utilized to monitor general characteristics of coral reefs and their associated populations. The technique, which has been employed in both scientist-run and community based programs, consists of towing a snorkeler trained to observe certain variables behind a boat at constant speed along a pre-determined stretch of reef (Bass and Miller 1996, Uychiaoco et al. 2005). In one study along the Great Barrier Reef, where manta tows have been employed since the 1970s, the sampled line is broken up into zones that take two minutes to sample and every two minutes, the boat stops for the observer to record data on an aquatic data sheet (Bass and Miller 1996). In these surveys, data often include counts of conspicuous species, such as giant clams, or of entire assemblages, such as carnivorous and herbivorous fish, but the technique is also used for monitoring habitat (Bass and Miller 1996, Uychiaoco et al. 2005). Indeed, observations of suites of variables designed to inform practitioners about the state of coral reefs over time such as reef slope, dominant benthic form, dominant hard coral genus, and structural complexity of coral are also commonly recorded (Bass and Miller 1996, Uychiaoco et al 2005).
Welsh et al. (1987) proposed a habitat-based approach for amphibians in small headwater streams and time-constrained and area-constrained approaches have also been used for headwater species (Hossack et al. 2006). Pond-breeding species or species that inhabit deeper water are often sampled using minnow traps, nets, or call counts of vocalizing frogs and toads (Kolozsvary and Swihart 1999, Crouch and Paton 2002).
Tracking of individual animals through tags, passive integrated transponders (PIT tags) and similar techniques are expensive but provide information on animal movements and estimates of population size and survival. Such approaches have been used with species of high interest such as coho salmon in the Pacific Northwest (Wigington et al. 2006).
Terrestrial and Semi-Aquatic Organisms
The diversity of forms, sizes, and life histories among terrestrial vertebrates has led to the development of hundreds of field techniques designed for different species and survey conditions. Table 8.1 lists the most widely used field techniques for collecting wildlife data, but it is by no means an exhaustive list of all inventory and monitoring methods. Techniques are separated into observational, capture, and marking methods and by the mode by which data are collected. A comprehensive review of all the different field techniques for terrestrial and semi-aquatic organisms is a separate book in itself (see Bookhout 1994). Here we provide a brief overview of some of the commonly used techniques.
Kuenzi and Morrison 1998 TagsBirds/MammalsNietfeld et al. 1994
Mode | Technique | Target Groups of Species | References | |
Observational | Direct | Quadrats; fixed-area plots | Sessile or relatively immobile organisms | Bonham 1989 |
Avian point counts | Bird species that sing or call on territories | Ralph et al. 1995 | ||
Spot mapping & nest searches | Territorial bird species | Ralph et al. 1993 | ||
Line transect | Large mammals, birds | Anderson et al. 1979 | ||
Call playback response | Wolves, ground squirrels, raptors, woodpeckers | Ogutu and Dublin 1998 | ||
Standardized visual searches | Large herbivores, | Cook and Jacobsen 1979 | ||
Census | Cave-dwelling bats; large herbivores | Thomas and West 1989 | ||
Animal
Sign |
Foot track surveys | Medium-large mammals | Wilson and Delahay 2001 | |
Pellet & scat counts | Medium-large mammals | Fuller 1991 | ||
Food cache searches | Large carnivores | Easter-Pilcher 1990 | ||
Structures (e.g., dens, nests) | Arboreal mammals; fossorial mammals; bears | Healy and Welsh 1992 | ||
Remote Sensing | Track plates | Medium-large mammals | Wilson and Delahay 2001 | |
Photo & video stations | Medium-large mammals | Morruzzi et al. 2002 | ||
Ultrasonic detectors | Bats | Thomas and West 1989 | ||
Audio monitoring | Frogs | Crouch and Paton 2002 | ||
Hair traps | Small-medium mammals, large carnivores | McDaniel et al. 2000 | ||
Radio telemetry | Limited by animal body size (>20 g) ? | USGS 1997 | ||
GPS telemetry | Limited by animal body ( >2000 g) ? | Girard et al. 2002 | ||
Marine radar | Bats, migrating birds | Harmata et al. 1999 | ||
Harmonic radar | Bats, amphibians, reptiles | Pellet et al. 2006 | ||
Capture | Passive | Pitfalls | Salamanders, lizards, small mammals | Enge 2001, Mengak and Guynn 1987 |
Snap traps | Small mammals | Mengak and Guynn 1987 | ||
Box traps | Small-medium mammals | Powell and Proulx 2003 | ||
Funnel-type traps | Snakes, turtles | Enge 2001 | ||
Leg-hold & snares | Large mammals | Bookhout 1994 | ||
Mist nets | ||||
Active | Drives to an enclosure | Medium-large mammals with predictable flight response | deCalesta and Witmer 1990 | |
Cannon nets | Medium-large mammals | Bookhout 1994 | ||
Immobilizing agents | Large mammals | Bookhout 1994 | ||
Hand capture | Salamanders | Kolozsvary and Swihart. 1999 | ||
Marking | ||||
Mutilation | Small mammals | Wood and Slade 1990 | ||
Pigments | Small mammals | Lemen and Freeman 1985 | ||
Collars & Bands | Birds/mammals | Nietfeld et al. 1994 |
For certain species and conditions, it may be feasible to determine a count of individual members of the population on quadrats (sample plots) randomly or systematically positioned in the study area. Searches can be conducted on foot, all-terrain vehicles, or airplane depending on the scale and circumstances of the survey. Quadrat sampling is commonly used for plants and habitat elements, but with animals quadrat sampling poses some challenges. If animals are mobile during the sampling period, then there needs to be some reasonable assurance that an individual is not double-counted at multiple quadrats as it moves. Size, spacing, and mobility of the organisms must all be considered.
Point counts are perhaps the most extensively used technique for measuring bird abundance and diversity in temperate forests and on rangelands but have also been used to estimate abundance of other diurnal species such as squirrels. Variations in the technique have been described for different species and to meet different data needs (Verner and Ritter 1985, Verner 1988, Ralph et al. 1995, Huff et al. 2000). Ralph et al. (1995) provided a collection of papers examining sample size adequacy, bird detectability, observer bias, and comparisons among techniques.
Spot mapping, also referred to as territory mapping, often is used to estimate avian population densities by locating singing males during a number of visits to the study area and delineating territory boundaries. The technique is further described in Ralph et al. (1995). Nest searches can be used to assess reproductive success in an avian population by monitoring the survival of eggs and nestlings over the course of a breeding season. Both techniques are labor intensive and are not commonly used for inventories, but the information gained from these methods (i.e., territory densities, productivity) may be better indicators of population trends and habitat quality than simply counts of individuals.
Line transect and point transect sampling are specialized plot methods in which a search for the target organism is conducted along a narrow strip having a known area. Rarely can it be assumed that all animals are detected along the transect. However, if the probability of detection can be predicted from the distance between the animal and the centerline of the transect, then a detection function can be used to estimate population density. The approach can be adapted to surveys conducted by foot, snorkeling, and ground or air vehicles. Buckland et al. (1993) provided a complete, though highly technical, introduction to line transect and point transect methods. The approach has been widely applied to surveys of vertebrates, including desert tortoise (Anderson et al. 2001), marbled murrelets (Madsen et al. 1999), songbirds in oak-pine woodlands (Verner and Ritter 1985), and mule deer (White et al. 1989).
Audio recordings of animal vocalizations have been used to elicit calls and displays from species otherwise difficult to detect. The technique has been applied in studies of blue grouse (Stirling and Bendell 1966), northern spotted owls (Forsman 1988), ground squirrels (Lishak 1977), and others. The number of responses by the target species elicited by the recording is tallied during a prescribed interval and provides a population density index.
Standardized visual searches refer to techniques used to determine species occurrence, species richness, or relative density values, where sampling effort is standardized by space or time. Examples include road counts for large mammals (Rudran et al. 1996), raptor migration counts (Hussell 1981), and visual encounter surveys for terrestrial amphibians (Crump and Scott 1994) (Figure 8.2). Some visual search techniques do not necessarily equalize the amount of survey effort among sampling units. Instead, animal counts or species detected are standardized during analysis by dividing the number of observations by a unit of area or time. Variability among observers and environmental conditions may be significant sources of error unassociated with the sampling technique and should be assessed prior to data collection to minimize biases and improve precision.
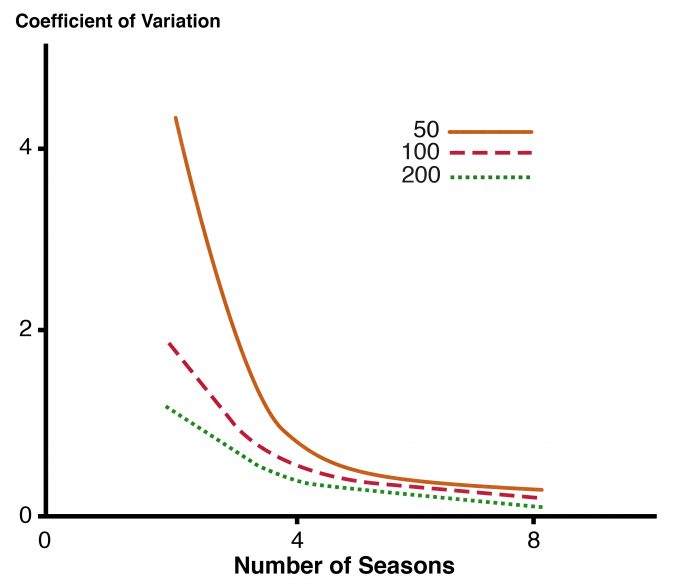
Under certain circumstances, it may be possible to effectively observe all individuals in the target population. In such cases, population size can be determined directly from the count of individuals; no statistical procedures are necessary. Accurate counts on individuals depend upon a natural tendency of population members to aggregate, at least during predictable periods (e.g., cave-roosting bats). Furthermore, all locations where the individuals aggregate must be known in the study area, and there must be adequate surveyors available to make simultaneous counts at all locations.
In many cases, the target population is highly cryptic or too wary to be observed directly and budget constraints prevent the investigator from utilizing capture methods for data collection. In these situations, it is often possible to infer the presence of a species or determine an index value for population density by observing animal signs. Signs are tracks, scat piles, fecal pellets, scent-marking posts, or animal constructions (e.g., arboreal nests, beaver lodges, burrow openings) that can be accurately identified as evidence of a particular species. If searches for such evidence are conducted on standardized transects or quadrats, then observations may provide a reliable index to population density. Data analyses are similar to that for direct observations. Davis and Winstead (1980) and Wemmer et. al (1996) provide an overview of methods based on animal sign.
Elusive species can be sampled using remote sensing devices positioned across the study area. Track plates (Zelinski and Stauffer 1996) and hair traps (Scotts and Craig 1988, McDaniel et al. 2000) are inexpensive and suitable for determining the occurrence and distribution of rare mammals in the study area. Ultrasonic detectors can be used to monitor bat populations (Kunz et al. 1996), however it is not always possible to reliably distinguish among all bat species. Remote camera stations with data-loggers (Cutler and Swann 1999, Moruzzi et al. 2002) not only detect occurrence of the species, but also may yield information about sex, age, and activity patterns of individuals. Baits at a track plate or camera station can be used to increase the probability on detecting a cryptic or rare species, but can also bias any estimates made or disproportionately attract common omnivorous species, such as Virginia opossum or northern raccoon (Figure 8.3).
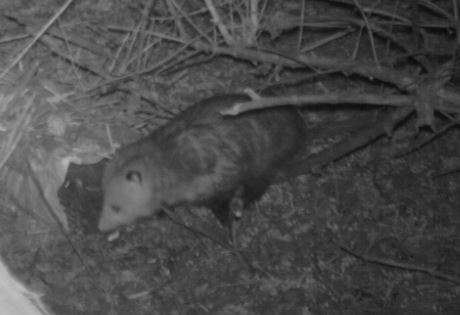
Radio-telemetry has been used for many years to collect data on wildlife and fish movement, home range size, and habitat selection (Figure 8.4). Transmitters weighing <1.0 g are now commercial available making it possible to track all but the smallest vertebrates. Tracking systems utilizing global positioning system (GPS) satellites permit monitoring of animal locations in real-time without requiring surveyors to determine radio signal directions in the field and are becoming more reasonably priced. A collection of abstracts on wildlife telemetry methods (USGS 1997) provides a useful introduction to the topic for terrestrial studies. Marine band radar has been used to count migratory birds at observation points (Harmata et al. 1999) and monitor activity patterns of marbled murrelets (Burger 2001).
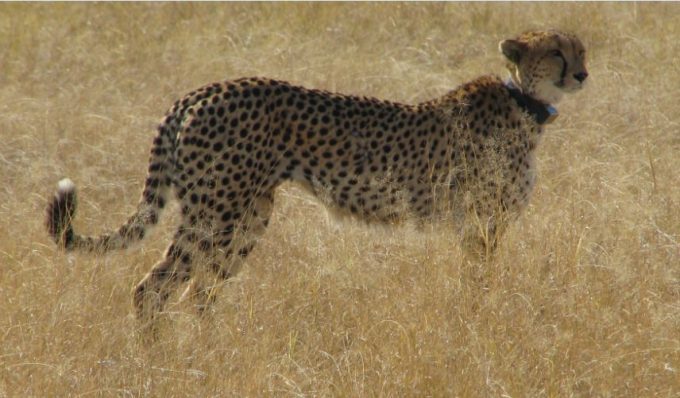
Finally genetic analyses of tissue collected from hair traps, scat or other tissues have led to an explosion of approaches to assess populations, dispersal, and evolutionary patterns (Haig 1998, Mills et al. 2000).
Life History and Population Characteristics
Certain techniques are more commonly used with some taxonomic groups than others. In this section we provide guidance as to the types of techniques that you might consider depending on the species included in your monitoring program.
Amphibians and Reptiles
The small size, cryptic nature, and fossorial habits of salamanders make data collection particularly difficult. Many terrestrial amphibians move only short distances and are not susceptible to passive capture techniques. These species are usually sampled using visual searches and hand capture techniques with sampling effort standardized by area (Jaeger and Inger 1994, Bailey et al. 2004) or a time constraint (Crump and Scott 1994) (Figure 8.2). Species that migrate between aquatic breeding ponds and terrestrial habitat types may be susceptible to pitfall traps with drift fences (Corn 1994). For pond-breeding species, egg masses are often more detectable than adults of the same species, making egg masses more suitable for population monitoring studies. Shaffer et al. (1994) and Olson et al. (1997) provided an excellent introduction to techniques for amphibian inventories in ponds.
Cover-board surveys have been widely adopted for estimating the relative abundance of amphibian and reptile populations in different habitat types (Grant et al. 1992, Harpole and Haas 1999, Engelstoft and Ovaskake 2000). Cover-boards are objects such as boards or metal roofing that provides daytime cover for animals and when lifted reveals a sample of the animals in the area that use it (Figure 8.5). Hence it is a sample of a plot for those species that seek cover.
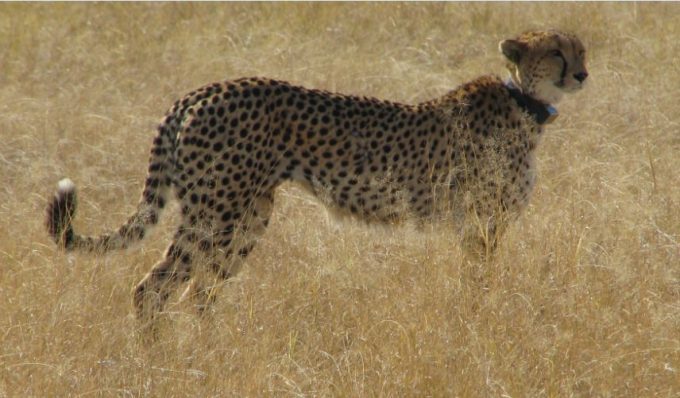
Birds
As a group, many species of birds are sampled using distance sampling because they are so mobile and vocal (Rostenstock et al. 2002, and see Buckland et al. 1993 for an overview of distance sampling). Consequently there are two primary means of detecting many species of birds, increasing the likelihood that they may be detected, especially during the breeding season when males are often territorial. Diurnal species sampled in grasslands, marshes or other rather uniform vegetation conditions often can be sampled using transects (e.g., Ribic and Sample 2001). Samples taken in areas where rugged terrain or other factors prevent the use of transects rely on point counts (Buckland 1987). Other commonly used techniques include spot mapping to understand territory densities (e.g., Dobkin and Rich 1998), nest searches to understand nest densities, and variations on the Mayfield method of calculating nest success (Johnson and Shaeffer 1990). Nocturnal species present additional challenges, but point counts for owls during their mating season can be effective especially if call back recordings are used to elicit responses (Hardy and Morrison 2000). But call back recordings can introduce biases when recordings are played in areas having different vegetative structure or topography. Finally, banding and band returns can be used to estimate longevity and age structures of populations (Pollock and Raveling 1982).
Mammals
Small mammals are often sampled using traps of various forms (Figure 8.6). Live traps are often used as they are suitable for mark-recapture estimates of population size, or live or kill traps can be used to estimate catch per unit effort estimates of relative abundance. When any kind of trap is used, animal welfare guidelines should be reviewed and followed.
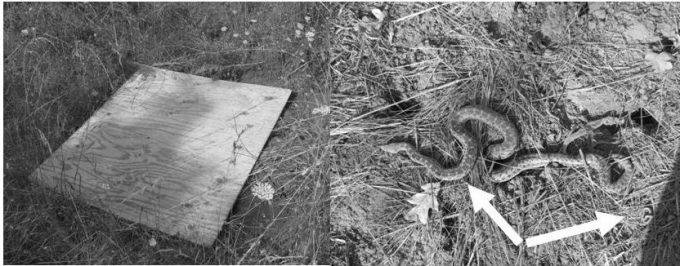
Detection probabilities are influenced by the number of trap-nights (the number of traps multiplied by the number of nights sampled). Capture rates are easily influenced by factors such as density-dependent intra-specific interactions, weather, and habitat, so capture probabilities must be calculated to allow an unbiased estimate of relative abundance (Menkens and Anderson 1988). This is particularly important when assessing trends over time during circumstances where weather and other conditions affecting catchability vary from year to year.
Larger mammals, especially those that form social aggregations or occur as clustered populations may be more effectively surveyed by observational techniques than capture methods. Aerial surveys for ungulates are often conducted using distance sampling procedures, but again observability among vegetation types, weather conditions, and topographies need to be considered to ensure unbiased estimates of abundance (Pollock and Kendall 1987).
Occurrence and indices to abundance for mid-size mammals are often addressed using remotely activated cameras, scent stations, track counts or track plates, or spotlight surveys (Figure 8.3; Gese 2001). Each of these techniques has advantages of being reasonably low cost and effective at detecting certain species (see Gese 2001), but estimation of population size is usually not possible.
Bats present a unique sampling problem. Using ultrasonic recordings of their feeding calls can help in distinguishing some species occurring in an area (O’Farrell et al. 1999), but estimates of abundance are often conducted at roost or maternity sites.
Effects of Terrain and Vegetation
Imagine that you are counting all the birds that you can see or hear along a transect that extends from the center of a forested patch to the center of an adjacent field. You wish to determine if the relative abundance of birds differs between the two habitat types. You detect 28 birds in the forest and 34 in the field. Are there more birds in the field than in the forest? Perhaps. But probably not. Your ability to detect birds in the forest is hampered by the decreased visibility in the forest compared to a more open field. Hence if you corrected for the differences in detectability as a function of the vegetation type (using techniques common in distance sampling) then the number of birds detected per unit area might be much greater in the forest than in the field. Consequently simply raw counts of animals without considering the distance from the observer to the animal are often biased and should not be used as response variables in monitoring programs. This is particularly important where vegetation structure is likely to change over the course of the monitoring time frame.
On human time-scales, terrain does not (usually) change appreciably from one time period to another, but observations must still be standardized by their detectability in various settings.
Merits and Limitations of Indices Compared to Estimators
Indices are often used to assess changes in populations over time based on an assumption that some aspect of detection is related to actual animal density. For instance, the following are a few examples of indices to abundance:
- track counts (Conner et al. 1983)
- pellet group counts (Fuller 1991)
- capture rates (Cole et al. 2000)
- detection rates of singing male birds (McGarigal and McComb 1998)
- relative dominance (plant populations)
- counts of squirrel leaf nests (Healy and Welsh 1992)
- counts of beaver lodges or caches (Easter-Pilcher 1990)
These examples do not provide estimates of populations, rather, an index is measured with the assumption that the index is related to the population or its fitness in a known manner and that observed changes in the index measurement over time will reflect changes in population according to this relationship. Assumptions such as the more tracks seen, the more individuals present; or the more male birds heard singing, the more birds reproducing at the site are often made. But the reliability of these assumptions is brought into question, and indeed the opportunity for bias associated with indices to abundance is quite high. For instance, track counts may be related to animal abundance or to animal activity levels, but on the other hand, they could be related to the characteristics of the substrate, the weather, or any combination of these options. Likewise, counts of singing male birds may represent trends in abundance of territorial males, but if some males do not attract a mate, then numbers of singing males may not indicate abundance of nesting females nor reproductive output. Capture rates of animals over space and time can be related to animal abundance or to their vulnerability to capture in different areas of habitat quality. Consequently, although indices to abundance are often used because of logistical constraints, considerable caution must be exercised when interpreting the results. Indeed, it is often useful to conduct a pilot study that will allow you to state with a known level of certainty what the relationship is between the index and the actual population (or fitness) for the species being monitored. To do so requires an estimate of the population.
Estimators provide additional information to the user and can help to address some of the biases inherent in many indices. Fortunately there are a number of tools available to estimate abundances that are based on sampling theory and can result in known levels of confidence placed around the estimates. For instance, distance estimators provide a mechanism for estimating abundance of organisms from points or transects where detectability might differ among vegetative types or stream reaches. Population estimators are generally available as free software: https://www.usgs.gov/software/wildlife-software-and-models
A variety of information is used as the basis for estimates of populations (capture-recapture and band returns), some of which can also provide estimates of survival and reproduction. The overwhelming advantage of using population estimators is that estimates of abundance, survival, and age class distribution can be made with estimates of confidence. Clearly with replicate sampling of independent sites, indices to abundance can be calculated with confidence intervals, but there is still doubt regarding the assumption of unbiased association between the index and the population characteristic of interest. Consequently it is important when designing monitoring protocols to ensure that estimators are considered, and if it is not logistically possible to use estimators as a response variable in the monitoring program, then the selected index should be justified relative to its known association with the population characteristic of interest. If this cannot be done, then both the assumptions and the implications of violating those assumptions should be clearly stated.
Estimating Community Structure
Although much of this book focuses on monitoring species and the environmental conditions in which they live, at times managers may be concerned with maintaining or developing conditions that promote diverse or functional communities, or be able to detect declines in functional diversity in the face of environmental stressors. Diversity metrics have evolved over decades and provide a means of comparing complexity between or among places or times. Unfortunately most diversity metrics also bury information on individual species responses within one or a few numbers. To understand what is really happening within a community, a diversity metrics must be deconstructed to see changes in individual species or populations. The devil is indeed in the detail. Nonetheless diversity metrics are still used as a guide to community structure and function. Typically, a diversity metric consists of an estimate of the number of species in a unit of space and time (species richness) and the distribution of individuals among those species (evenness). In most evenness metrics, the maximum is achievable value is 1.00 (an equal number of individuals per unit area represented among all species in the community; i.e., no species dominates the community). But consider the example in table 8.2. The hypothetical forest and grassland have the same diversity and evenness. So are they the same community? Do they function in a similar manner? Obviously not. In fact the degree to which the two communities are similar in species representation and distribution of individuals among species in common between the two communities indicates that the percent similarity between the two communities is 0. In fact, each community has more in common with a more species rich and more even community represented in a savannah than in the forest or grassland. So is the savannah better because it is more species rich and more diverse? No, it is just different. In short, these metrics of community structure can be very difficult to interpret without digging into the details that comprise the metrics.
Community | |||
Forest | Savannah | Grassland | |
Black-capped chickadee | 10 | 4 | 0 |
Song Sparrow | 2 | 4 | 0 |
American robin | 4 | 3 | 0 |
Grasshopper sparrow | 0 | 3 | 4 |
Marsh wren | 0 | 1 | 2 |
Savannah Sparrow | 0 | 1 | 10 |
___ | ___ | ___ | |
Species Richness | 3 | 6 | 3 |
Number of individuals | 16 | 16 | 16 |
Simpson’s Evenness | 0.797 | 0.956 | 0.797 |
Simpson’s Species Diversity1 | 0.567 | 0.850 | 0.567 |
Community similarity (%)2 | |||
Forest-Savannah | 0.563 | ||
Forest-Grassland | 0.000 | ||
Savannah-Grassland | 0.313 | ||
1 White (1986) | |||
2 Itow (1991) |
Sampling communities in a manner that produces unbiased estimates of community structure also can be problematic. Species richness assessments may be as simple as developing a list of species detected in an area using standardized techniques. But some species are likely more easily detected than others. Some are active at different times of the day, and some move more than others. So estimates of richness are confounded by differences in detectability. When diversity metrics are calculated using these data, additional complications arise because estimates of abundance for each species in the community must be unbiased and based on the same unit of space and time. Again, differential detectability of species and biases resulting from movement arise making evenness estimates confounded between actual differences in abundance among species and differences in detection among species. Surveys to determine the presence of a species in an area typically require less sampling intensity than fieldwork necessary to collect other population statistics.
Another metric that can be calculated from data collected within a monitoring framework deals with unequal sampling efforts when trying to estimate the number of species in an area. Because rare species are often less likely to be detected than common species, one can estimate the number of species based on rarefaction curves so that you can compare the number of species found in two areas when the sampling effort differed (Simberloff 1972).
Estimating biotic integrity
Karr (1981) developed and index to biotic integrity (IBI) that was designed to be used to compare aspects of fish communities among sites in a standardized manner that reflected the water quality of the site with regards to its capacity to support fish communities. It included aspects of species richness, fish health, and a number of other parameters. Researchers have since adapted this technique for other taxa including aquatic macroinvertebrates and birds (Bryce et al. 2002). Although the general structure of IBIs is similar to that developed by Karr (1981), each IBI is typically crafted to a reference condition typical of the ecoregion within which a sampled area occurs. Hence an IBI developed for the mixed mesophytic forest of the Appalachians may have a similar structure to that of one in the Cascades mountains of Oregon, but the parameters measured would be based on a very different set of reference conditions. For that reason IBIs are not typically used in terrestrial monitoring protocols and like diversity indices they can bury information that must be extracted by deconstructing the index.
Standardization Protocol Review
Regardless of the approach taken, certain aspects of a sampling design should be standardized to minimize bias in the resulting data. See chapter 7 for a more detailed overview of standardization techniques. At the very least specific attention should be paid to consistently sampling the same location over time, sampling during the same season and time of day and using the same equipment from one sampling period to the next.
In addition to consistency in sampling techniques, locations and times, most of the approaches described in this chapter will need approval by an Institutional Animal Care and Use Committee (IACUC) or comparable review board at universities and agencies. Standards of Care for animals when conducting research are rigorous and should be applied to monitoring programs as well (see Laber et al. 2007 and Hafner 2007 for a perspective on limitations to such reviews).
Budget Constraints
The choice of which sampling technique to use when presented with options and a set of goals and objectives is as often driven my constraints of time and money as by the ideal technique to acquire the data needed. For instance, while information on survival of juveniles in the face of climate change may be a primary consideration for a long-lived species, a suitable surrogate might be an index to abundance of young of the year and adult animals in the population. Since budget decisions are often made based on the social seriousness of the issue, and since social values change over time, so do budgets. Consequently it often is wise to develop a monitoring program using techniques that are robust to concerns regarding bias and precision and that are cost effective, even if they do not result in the ideal level of fitness data that would be needed to answer demographic questions more definitively. More detailed demographic information may be collected in a supplementary manner if the level of social concern reaches a point where it is mandated.
Summary
There is a huge array of methods available to detect, enumerate or assess the fitness of organisms. Matching the appropriate approach with the goals and objectives of the study is a key first step in designing a monitoring program. The data needed to achieve inventory and monitoring objectives is often the driving factor in deciding which techniques will be used in a monitoring program. In addition the scale of the monitoring program may also influence the methods used. For narrowly focused programs on highly valued species, detailed measurements of fitness may be appropriate. As the scale of the project over space and time increases, then estimates of abundance or occurrence may be the most feasible approach.
Furthermore, different taxa vary in their propensity to be sampled using different methods. Whereas sessile animals can be sampled using quadrat sampling, wide-ranging species may more easily be sampled using cameras or hair traps that may provide information on occurrence or individual movements. In a similar way, simply the logistics associated with sampling in inhospitable (to humans) terrain limits the choices of sampling strategies available. Finally, budgetary and logistic restrictions oftentimes mean that the ideal sampling system may not be feasible because the time or money is not available to implement the technique to adequately meet the goals and objectives.
Amidst all of the decisions regarding which technique to employ, you should continually be aware of the need to standardize approaches over space and time and seek to minimize any biases in your sampling methods.
References
Anderson, D.R., J.L. Laake, B.R.Cran, and K.P. Burnham. 1979. Guidelines for line transect sampling of biological populations. Journal of Wildlife Management 43: 70–78.
Anderson, D.R., K.P. Burnham, B.C. Lubow, L. Thomas, P.S. Corn, P.A. Medica, and R.W. Marlow. 2001. Field trials of line transect methods applied to estimation of desert tortoise abundance. Journal of Wildlife Management 65: 583–597.
Bailey, L.L., T.R.Simons, and K.H. Pollock. 2004. Estimating site occupancy and species detection probability parameters for terrestrial salamanders. Ecological Applications 14:692–702.
Bass, D.K. and I.R. Miller. 1996. Crown-of-thorns starfish and coral surveys using the manta tow and scuba search techniques. Long-term monitoring of the Great Barrier Reef standard operational procedure number 1. Australian Institute of Marine Science, Townsville.
Bonham, C. D. 1989. Measurements of terrestrial vegetation. John Wiley, New York, New York, USA. 338pp.
Bookhout, T.A. (ed). 1994. Research and management techniques for wildlife and habitats. The Wildlife Society, Bethesda, Maryland. 740 pp.
Bryce, S.A., R.M. Hughes, and P.R. Kaufman. 2002. Development of a bird integrity index: using bird assemblages as indicators of riparian condition. Environmental Management 30:294–310.
Buckland, S.T. 1987. On the variable circular plot method of estimating animal density. Biometrics 43:363–384.
Buckland, S.T., D.R. Anderson, K P. Burnham, and J.L. Laake. 1993. Distance Sampling: Estimating Abundance of Biological Populations. Chapman and Hall, New York. 446pp.
Burger, A.E. 2001. Using radar to estimate populations and assess habitat associations of Marbled Murrelets. Journal of Wildlife Management 65: 696–715.
Castello, L., J.P. Viana, G. Watkins, M. Pinedo-Vasquez, and V.A. Luzadis. 2009. Lessons from integrating fishers of Arapaima in small-scale fisheries management at the Mamirauá Reserve, Amazon. Environmental Management 43:197-209.
Cole, E.C., W.C. McComb, M, Newton, J.P. Leeming, and C.L. Chambers. 1998. Response of small mammals to clearcutting, burning, and glyphosate application in the Oregon coast range. Journal of Wildlife Management 62:1207–1216.
Conner, M.C., R.F. Labisky, and D.R. Progulske, Jr. 1983. Scent-station indices as measures of population abundance for bobcats, raccoons, grey foxes and opossums. Wildlife Society Bulletin 11, 146–152.
Cook, R.D., and J.O. Jacobson. 1979. A design for estimating visibility bias in aerial surveys. Biometrics 35:735–742.
Corn, P.S. 1994. Straight line drift fences and pitfall traps. Pages 109-117 In W.R. Heyer, M A. Donnelly, R.W. McDiarmid, L.C. Hayek, and M.S. Foster, editors. Measuring And Monitoring Biological Diversity: Standard Methods for Amphibians,. Smithsonian Instit. Press, Washington, D.C.
Crouch, W.B., and P.W.C. Paton. 2002. Assessing the use of call surveys to monitor breeding anurans in Rhode Island. Journal of Herpetology 36, 185–192.
Crump, M.L., and N.J. Scott Jr. 1994. Visual encounter surveys. Pages 84–92 In W.R. Heyer, M.A. Donnelly, R.W. McDiarmid, L.C. Hayek, and M.S. Foster, editors. Measuring and Monitoring Biological Diversity: Standard Methods for Amphibians,. Smithsonian Institution Press, Washington, DC.
Cunjak, R.A., R.G. Randall, and E.M.P. Chadwick. 1989. Snorkeling versus electrofishing: a comparison of census techniques in Atlantic salmon rivers. Naturaliste Canadien. 115: 89-93.
Cutler, T., and D.E. Swann. 1999. Using remote photography in wildlife ecology: a review. Wildl. Soc. Bull. 27:571-581.
Davis, D.E. and R.L. Winstead,. 1980. Estimating the numbers of wildlife populations. Pages. 221–245.In S.D. Schemnitz, editor, Wildlife management techniques manual, The Wildlife Society, Boston.
deCalesta, D.S. and G.W. Witmer. 1990. Drive-line census for deer within fenced enclosures. USDA Forest Service Research Paper NE-643. 4pp.
Dobkin D.S., and A.C. Rich. 1998. Comparison of line-transect, spotmap, and point-count surveys for birds in riparian areas of the Great Basin. Journal of Field Ornitholology 69:430–443
Easter-Pilcher, A. 1990. Cache size as an index to beaver colony size in northwestern Montana. Wildlife Society Bulletin 18:110–113.
Elzinga, C.L., D.W. Salzer and J.W.Willoughby. 1998. Measuring and monitoring plant populations. Bureau of Land Management, Technical Reference 1730–1, Denver, Colorado, USA.
Enge, K.M. 2001. The pitfalls of pitfall traps. Journal of Herpetology 35:467–478.
Engelstoft, C. and K.E. Ovaskake. 2000. Artificial cover objects as a method for sampling snakes (Contia tenuis and Thamnophis spp.) in British Columbia. Northwestern Naturalist 81:35–43.
Forsman, E.D. 1988. A survey of spotted owls in young forests in the northern Coast Range of Oregon. Murrelet 69:65-68.
Fuller, T.K. 1991. Do pellet counts index white-tailed deer numbers and population change? Journal of Wildlife Management 55, 393–396.
Gese, E.M., 2001. Monitoring of terrestrial carnivore populations. Pages 373–396 In: J.L. Gittleman et al., editors. Carnivore Conservation. Cambridge University Press, Cambridge, UK.
Girard, I., J.P. Ouellet, R. Courtois, C. Dussault, and L. Breton. 2002. Effects of sampling effort based on GPS telemetry on home-range size estimations. Journal of Wildlife Management 66:1290–1300.
Grant, B.W., A.D. Tucker, J.E. Lovich, A.T. Mills, P.M. Dixon, and J.W. Gibbons. 1992. The use of coverboards in estimating patterns of reptile and amphibian abundance. Pages 379–403 In R. Seigel and N. Scott, editors. Wildlife 2001, Elsevier Science Publ., Inc., London, UK.
Haig, S. 1998. Molecular contributions to conservation. Ecology 79:413–425.
Hafner, M.S. 2007. Field research in mammalogy: an enterprise in peril. Journal of Mammalogy 88:1119–1128.
Hankin, D.G., and G.H. Reeves. 1988. Estimating total fish abundance and total habitat area in small streams based on visual estimation methods. Canadian Journal of Fisheries and Aquatic Sciences 45:834–844.
Hardy, P.C. and M.L. Morrison. 2000. Factors affecting the detection of Elf Owls and Western Screech-Owls. Wildlife Society Bulletin 28:333–343.
Harmata, A.R., K.M. Podruzny, J.R. Zelenak, and M.L. Morrison. 1999. Using marine surveillance radar to study bird movements and impact assessment. Wildlife Society Bulletin 27:44–52.
Harpole, D.N. and C.A. Haas. 1999. Effects of seven silvicultural treatments on terrestrial salamanders. Forest Ecology and Management 114:349-356.
Healy, W.M. and C.J.E. Welsh. 1992. Evaluating line transects to monitor grey squirrel populations. Wildlife Society Bulletin 20:83–90.
Hossack, B.R., P.S. Corn, and D.B. Fagre. 2006. Divergent patterns of abundance and age-class structure of headwater stream tadpoles in burned and unburned watersheds. Canadian Journal of Zoology 84:1482–1488.
Huff, M.H., K.A. Bettinger, H.L. Ferguson, M.J. Brown, and B. Altman. 2000. A habitat-based point-count protocol for terrestrial birds, emphasizing Washington and Oregon. United States Forest Service General Technical Report PNW-GTR-501
Hussell, D.J.T. 1981. The use of migration counts for monitoring bird population levels. Pages 92–102 in C. J. Ralph and J. M. Scott , editors. Estimating Numbers of Terrestrial Birds. Studies in Avian Biology, no. 6.
Itow, S. 1991. Species turnover and diversity patterns along an evergreen broad-leaved forest coenocline. J. Veg. Sci. 2: 477-484.
Jaeger, R.G., and R.F.Iinger. 1994. Quadrat sampling. pp. 97–102 In W.R. Heyer, M.A. Donnelly, R.W. McDiarmid, L.C. Hayek, and M.S. Foster, editors. Measuring and monitoring biological diversity: Standard Methods for Amphibians, Smithsonian Institution Press, Washington, DC.
Johnson, D.H., and T.L. Shaffer. 1990. Estimating nest success: when Mayfield wins. Auk 107: 595–600.
Karr, J.R. 1981. Assessment of biotic integrity using fish communities. Fisheries 6:(6)21–27.
Kolozsvary M.B., and R.K. Swihart. 1999. Habitat fragmentation and the distribution of amphibians: patch and landscape correlates in farmland. Canadian Journal of Zoology 77:1288–1299
Kuenzi A.J. and M.L. Morrisson. 1998. Detection of bats by mist-nets and ultrasonic sensors. Wildlife Society Bulletin 26: 307-311.
Kunz, T.H., D.W. Thomas, G.C. Richards, C.R. Tidemann , E.D. Pierson, and P.A.
Racey. 1996. Observational techniques for bats. Pages 105–114 In: D.E. Wilson, F.R. Cole, J.D. Nichols, R. Rudran, and M.S. Foster, Editors. Measuring and Monitoring Biological Diversity. Smithsonian Institution Press, Washington, D.C.
Laber, K., B.W. Kennedy, and L. Young. 2007. Field studies and the IACUC: protocol review, oversight, and occupational health and safety considerations. Lab Animal 36:27–33.
Lande, R. and G.F. Barrowclough. 1987. Effective population size, genetic variation, and their use in population management. Pages 87-123 in: M.E. Soulé, Editor, Viable Populations for Conservation, Cambridge University Press. Cambridge, UK. 204pp.
Lemen, C.A., and P.W. Freeman. 1985. Tracking mammals with fluorescent pigments: a new technique. Journal of Mammalogy 66:134–136
Lint, J. 2001. Northern spotted owl effectiveness monitoring plan under the Northwest Forest Plan: Annual summary report 2000. Northwest Forest Plan Interagency Monitoring Program, Regional Ecosystem Office, Portland, OR.
Lishak, R. S. 1977. Censusing 13-lined ground squirrel with adult and young alarm calls. Journal of Wildlife Management 41:755–759.
MacKenzie, D.I. 2005. Was it there? Dealing with imperfect detection for species presence/absence data. Australia and New Zealand Journal of Statistics 47:65–74.
MacKenzie, D.I., J.D. Nichols, J.E. Hines, M.G. Knutson, and A.D. Franklin. 2003. Estimating site occupancy, colonization and local extinction probabilities when a species is not detected with certainty. Ecology 84:2200–2207.
MacKenzie, D.I., and J.A. Royle. 2005. Designing efficient occupancy studies: general advice and tips on allocation of survey effort. Journal of Applied Ecology 42:1105–1114.
Madsen, S., D. Evans, T. Hamer, P. Henson, S. Miller, S.K. Nelson, D. Roby, and M Stapanian. 1999. Marbled murrelet effectiveness monitoring plan for the Northwest Forest Plan. USDA Forest Service General Technical Report PNW-GTR-439. 51 pp.
McDaniel, G.W., K.S. McKelvey, J.R. Squires, and L.F. Ruggiero. 2000. Efficacy of lures and hair snares to detect lynx. Wildlife Society Bulletin 28:119–123.
McGarigal, K. and W.C. McComb. 1999. Forest fragmentation and breeding bird communities in the Oregon Coast Range. Chapter 13 in J. P. Rochelle, L. A. Lehman, and J. Wisniewski, editors. Forest fragmentation: Wildlife and Management Implications. Brill Press, Netherlands.
Mengak, M.T., and D.C. Guynn. 1987. Pitfalls and snap traps for sampling small mammals and herpetofauna. American Midland Naturalist 118:284–288.
Menkens, G.E., and S.H. Anderson. 1988. Estimation of small-mammal population size. Ecology 69:1952–1959.
Mills L.S., J.J. Citta, K.P. Lair, M.K. Schwartz, and D.A. Tallmon. 2000. Estimating animal abundance using noninvasive DNA sampling: promise and pitfalls. Ecological Applications 10:283–294.
Moruzzi,T.L., T.K. Fuller, R.M. DeGraaf, R.T. Brooks,and W.J. Li. 2002. Assessing remotely triggered cameras for surveying carnivore distribution. Wildlife Society Bulletin 30:380-386
Nichols, J.D., J.E. Hines, J.R. Sauer, F.W. Fallon, J E. Fallon, and P.J. Heglund. 2000. A double observer approach for estimating detection probability and abundance from point counts. Auk 117:393–408.
Nietfeld, M. T., M. W. Barrett, and N. Silvy. 1994. Wildlife marking techniques. Pages 140-168 In T. A. Bookhout, editor. Research and management techniques for wildlife and habitats. The Wildlife Society, Bethesda, Md.
O’Farrell, M.J., B.W. Miller, and W.L. Gannno.1999. Qualitative identification of free-flying bats using the Anabat detector. Journal of Mammalogy 80:11–23.
Ogutu, J.O. and H.T. Dublin. 1998. The response of lions and spotted hyaenas to sound playbacks as a technique for estimating population size. African Journal of Ecology 36: 83–95.
Olson, D.H., W.P. Leonard, and R.B. Bury. 1997. Sampling amphibians in lentic habitats: methods and approaches for the Pacific Northwest. Society for Northwestern Vertebrate Biology, Olympia, Wash.
Pellet, J., L. Rechsteiner, A.K. Skrivervik, J.F. Zürcher, and N. Perrin. 2006. Use of harmonic direction finder to study the terrestrial habitats of the European tree frog (Hyla arborea). Amphibia-Reptilia 27:138-142.
Pelletier, F., D. Reale, J. Watters, E. H. Boakes, and D. Garant. 2009. Value of captive populations for quantitative genetics research. Trends in Ecology and Evolution 24:263-270.
Poizat, B. and E. Baran. 1997. Fishermen’s knowledge as background information in tropical fish ecology: a quantitative comparison with fish sampling results. Environmental Biology of Fishes. 50(4):435-449.
Pollock, K.H., and D.G. Raveling. 1982. Assumptions of modern band-recovery models, with emphasis on heterogeneous survival rates. Journal of Wildlife Management 46:88–98.
Pollock, K.H., and W.L. Kendall. 1987. Visibility in aerial surveys: A review of estimation procedures. Journal of Wildlife Management 51:502–510.
Powell, R.A. and G. Proulx. 2003. Trapping and marking terrestrial mammals for research: integrating ethics, performance criteria, techniques, and common sense. Ilar Journal 44:259–276.
Pradel, R. 1996. Utilization of capture-mark-recapture for the study of recruitment and population growth rates. Biometrics 52 703-709.
Ralph, C.J., S. Droege, and J.R. Sauer. 1995. Managing and monitoring birds using point counts: Standards and applications. Pages 161–168 in C. J. Ralph, J. R. Sauer, and S. Droege, Editors. Monitoring Bird Populations by Point Counts.USDA Forest Service General Technical Report PSW-GTR-149.
Ralph, C.J., G.R. Geupel, P. Pyle, T.E. Martin, and D.F. DeSante. 1993. Handbook of field methods for monitoring landbirds. USDA Forest Service General Technical Report PSW-GTR-144. 41pp.
Ribic, C.A., and D.W. Sample. 2001. Associations of grassland birds with landscape factors in southern Wisconsin. American Midland Naturalist 146:105–121.
Rosenstock, S.S., D.R. Anderson, K.M. Giesen, T. Leukering, and M.F. Carter. 2002. Landbird counting techniques: Current practices and an alternative. Auk 119:46–53.
Rudran, R, T.H. Kunz, S.C. Jarman, and A.P. Smith. 1996. Observational techniques for nonvolant mammals. Pages 81–114 In D.E. Wilson, F.R. Cole, J.D. Nichols, R. Rudran, and M.S. Foster, Editors. Measuring and monitoring biological diversity. Standard methods for mammals. Smithsonian Institution, Washington, DC, USA.
Sauer, J.R., J.E. Hines, and J. Fallon. 2008. The North American Breeding Bird Survey, Results and Analysis 1966 – 2007. Version 5.15.2008. USGS Patuxent Wildlife Research Center, Laurel, MD
Scotts, D.J., and S.A. Craig, S.A. 1988. Improved hair-sampling tube for the detection of rare mammals. Australian Wildlife Research 15:469–472.
Seber, G.A.F. 1982. The Estimation of Animal Abundance, 2nd ed. Griffin Publishers, London. 654pp .
Shaffer H.B., R.A. Alford, B.D. Woodward, S.J. Richards, R.G. Altig, and C. Gascon. 1994. Quantitative sampling of amphibian larvae. Pages 130–141 In: Heyer W.R., M.A. Donnelly, R.W. McDiarmid, L.C. Hayek, and M.S. Foster., editors. Measuring and Monitoring Biological Diversity: Standard Methods for Amphibians. Smithsonian Institute Press, Washington, D.C.
Simberloff, D.S. 1972. Properties of the rarefaction diversity measurement. American Naturalist 106:414-418.
Simberloff, D.S. 1978. Use of rarefaction and related methods in ecology. pp. 150-165 In K.L. Dickson, J. Cairns, Jr., and R.J. Livingston, Editors. Biological Data in Water Pollution Assessment: Quantitative and Statistical Analyses. American Society for Testing and Materials STP 652, Philadelphia, PA.
Slack, K.V., R.C. Averett, P.E. Greeson, and R.G. Lipscomb. 1973. Methods for collection and analysis of aquatic biological and microbiological samples: U.S. Geological Survey Techniques of Water-Resources Investigations. Report Number 05-A4.
Stirling, D. F, and J. F. Bendell. 1966. Census of Blue Grouse with recorded calls of the female. Journal of Wildlife Management 30:184–187.
Suckling, K. and M. Taylor. 2006. Critical habitat and recovery. Pages 50-67 in D.D. Goble, J.M. Scott and F.W. Davis. The Endangered Species Act at 30: Vol. 1: Renewing the Conservation Promise. Island Press. Washington, D.C.
Thomas, D.W., and S.D. West. 1989. Sampling methods for bats. USDA Forest Service General Technical Report PNW 243. 20pp.
U.S. Geological Survey. 1997. Forum on Wildlife Telemetry: Innovations, evaluations, and Research Needs; 21-23 September 1997, Snowmass Village, Colorado. Program and Abstracts. U.S. Geological Survey and The Wildlife Society.
Uychiaoco, A.J., H.O. Arceo, S.J. Green, M.T. De la Cruz, P.A. Gaite, and P.M. Aliño. 2005. Monitoring and evaluation of reef protected areas by local fishers in the Philippines: tightening the adaptive management cycle. Biodiversity and Conservation 14:2775-2794.
Verner, J., and L.V. Ritter. 1985. A comparison of transects and point counts in oak–pine woodlands of California. Condor 87:47–68.
Verner, J. 1988. Optimizing the duration of point counts for monitoring trends in bird populations. USDA Forest Service Research Note PSW-395.
Welsh, H.H., Jr. 1987. Monitoring herpetofauna in woodland habitats of northwestern California and southwestern Oregon: a comprehensive strategy. Pages 203-213 in T.R Plumb and N.H. Pillsbury, editors. Multiple-use management of California’s hardwood resources. USDA Forest Service General Technical Report PSW-100.
Wemmer C., T.H. Kunz, G. Lundie-Jenkins, and W. McShea. 1996. Mammalian sign. Pages 157-176 In D.E. Wilson, F.R. Cole, J.D. Nichols, R. Rudran, and M.S. Foster Editors. Measuring and Monitoring Biological Diversity – Standard Methods for Mammals. Smithsonian Institution Press, Washington, DC.
White, G.C., R.M. Bartmann, L.H. Carpenter, and R.A. Garrott. 1989. Evaluation of aerial line transects for estimating mule deer densities. Journal of Wildlife Management 53:625–635.
White, M.J. 1986. Segregation and diversity measures in population distribution. Population Index 52:198–221.
Wigington, P.J., J.L. Ebersole, M.E. Colvin, S.G. Leibowitz, B. Miller, B. Hansen, H. Lavigne, D. White, J.P. Baker, M.R. Church, J.R. Brooks, M.A. Cairns, and J.E. Compton. 2006. Coho salmon dependence on intermittent streams. Frontiers in Ecology and the Environment 4:513-518.
Wilson, G.J. and R.J. Delahay. 2001. A review of methods to estimate the abundance of terrestrial carnivores using field signs and observation. Wildlife Research 28:151–164.
Wood, M.D. and N.A. Slade. 1990. Comparison of ear-tagging and toe clipping in prairie voles, Microtus ochrogaster. Journal of Mammalogy 71: 252–255.
Zielinski, W.J. and H.B. Stauffer. 1996. Monitoring Martes populations in California: Survey design and power analysis. Ecological Applications 6: 1254–1267.