Main Body
One-Carbon Metabolism Micronutrients
Three B vitamins are involved in what is known as 1-carbon metabolism. This is the movement of 1 carbon units, generally methyl groups (CH3). It is similar to the movement of the amino group that occurs in transamination. As shown in the figure below, folate, vitamin B12, and vitamin B6 are the B vitamins involved in 1-carbon metabolism.
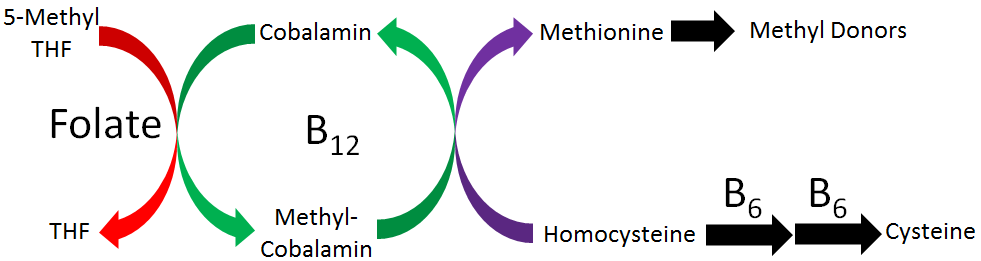
Vitamin B6 has been covered already in the previous chapter, so this chapter is going to focus on folate and vitamin B12. We will examine this figure in pieces, so that hopefully by the time this chapter is completed, you will understand the role of all these vitamins in 1-carbon metabolism.
Sections:
11.1 Folate & Folic Acid
11.2 Vitamin B12
11.3 B Vitamins, Homocysteine, & Cardiovascular Disease
11.1 Folate & Folic Acid
Folate is a B vitamin that exists in either its reduced form (folate) or oxidized form (folic acid). When folate is used in this section, we are referring to the reduced form, not the vitamin itself. Another key distinction between the 2 terms is that folic acid refers to the synthetic form, while folate refers to the natural form. Folic acid is only found in certain foods because they have been fortified with it, not because they produce it. The structure of folic acid is shown below.
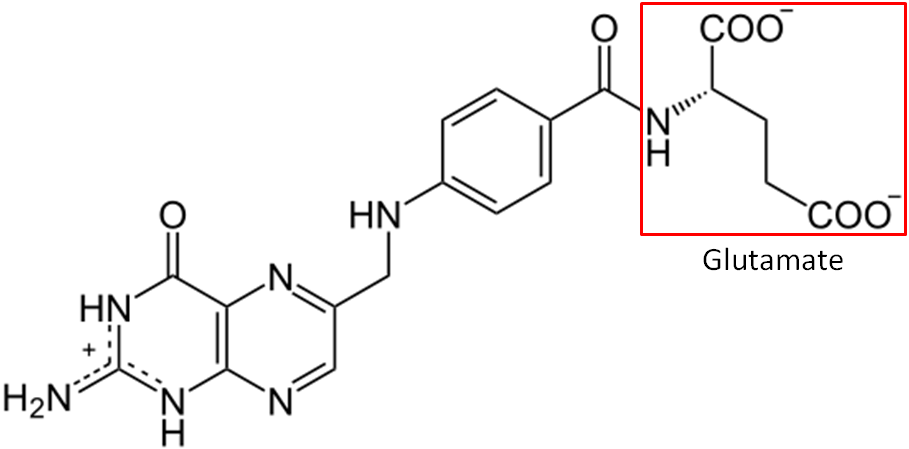
Another key difference between folate and folic acid is the number of glutamates in their tails. Notice that glutamate is boxed in the structure of folic acid above. Folic acid always exists as a monoglutamate, meaning it only contains 1 glutamate. On the other hand, about 90% of the folate found in foods are polyglutamates, meaning there is more than 1 glutamate in their tail. Folic acid is more stable than folate, which can be destroyed by heat, oxidation, and light2. Table 11.11 summarizes the key differences between folate and folic acid.
Table 11.11 Comparison of folate to folic acid
Folate | Folic Acid |
Reduced Form | Oxidized Form |
Natural | Synthetic |
Polyglutamate | Monoglutamate |
More Stable |
The bioavailability of folate was believed to be much lower than folic acid.3 To account for these differences, the DRI committee created dietary folate equivalents (DFEs) to set the DRIs4. DFEs are defined as follows:
1 DFE = 1 ug food folate = 0.6 ug food folic acid = 0.5 ug folic acid on an empty stomach
DFE = ug food folate + (ug folic acid X 1.7)
The 1.7 came from research suggesting that folic acid from food was 85% bioavailable, compared to 50% for folate (85%/50% = 1.7)4. This was established in 1998 by the DRI committee, and it is likely that these conversions & the requirements will change based on the newer evidence suggesting folate’s bioavailability from food is higher (80% of folic acid) than previously believed3. With this data, the new conversion factor for folic acid would be 1.25 (100%/80%). This conversion factor means that food folate levels are probably contributing more towards our dietary needs than currently being estimated by the DFE, but the DRI for folate/folic acid has not been updated.
Before folate (polyglutamates) can be taken up into the enterocyte, the extra glutamates must be cleaved prior to uptake into the enterocyte by the reduced folate transporter (RFT, aka reduced folate carrier)5-7. Folic acid, because it is a monoglutamate, requires no cleavage for uptake before it is taken up through the RFT. Once inside the enterocyte, the monoglutamate form is methylated and transported into circulation through an unresolved carrier5. This series of events is depicted in the figure below.
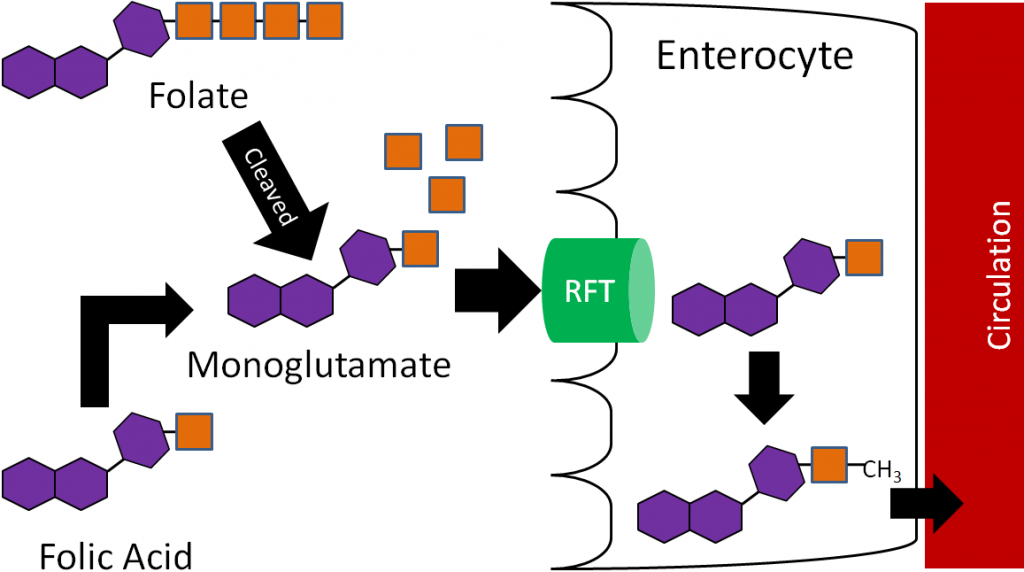
Thus, the methylated monoglutamate form is the circulating form. This is transported to the liver where it is converted back to the polyglutamate form for storage. Folate is excreted in both the urine and feces5.
Subsections:
11.11 Folate Functions
11.12 Folate Deficiency & Toxicity
References & Links
- http://en.wikipedia.org/wiki/File:Folat.svg
- Byrd-Bredbenner C, Moe G, Beshgetoor D, Berning J. (2009) Wardlaw’s perspectives in nutrition. New York, NY: McGraw-Hill.
- Winkels R, Brouwer I, Siebelink E, Katan M, Verhoef P. (2007) Bioavailability of food folates is 80% of that of folic acid. Am J Clin Nutr 85(2): 465-473.
- Anonymous. (1998) Dietary reference intakes for thiamin, riboflavin, niacin, vitamin B6, folate, vitamin B12, pantothenic acid, biotin, and choline. Washington D.C.: National Academies Press.
- Shils ME, Shike M, Ross AC, Caballero B, Cousins RJ, editors. (2006) Modern nutrition in health and disease. Baltimore, MD: Lippincott Williams & Wilkins.
- Gropper SS, Smith JL, Groff JL. (2008) Advanced nutrition and human metabolism. Belmont, CA: Wadsworth Publishing.
- Stipanuk MH. (2006) Biochemical, physiological, & molecular aspects of human nutrition. St. Louis, MO: Saunders Elsevier.
11.11 Folate Functions
The major function of folate is that it participates in 1-carbon metabolism. As described earlier, this is the transfer of 1-carbon units from 1 compound to another. The cofactor form of folate is tetrahydrofolate (THF). As is shown in the figure below, in order for THF to be formed, a methyl group is transferred to cobalamin (vitamin B12) from 5-methyl THF (THF plus a methyl group), forming methyl-cobalamin. You can see this on the left side of the figure below.
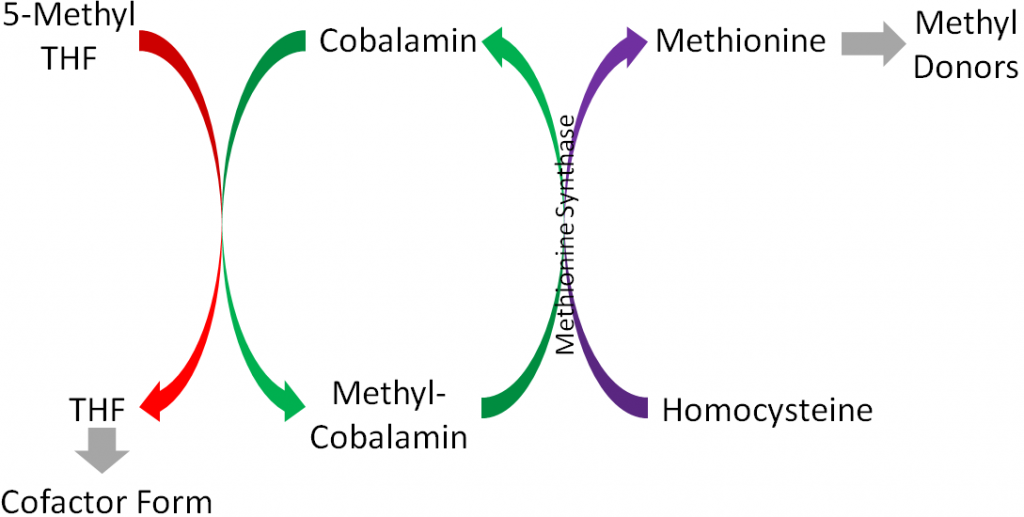
There are 2 major cofactor functions of THF1:
- DNA Synthesis
THF is required for the synthesis of DNA bases (purines and pyrimidines)1. As shown in the link below, N10-formyl-THF (a form of THF) is needed in 2 reactions (3 and 9) in purine synthesis.
Web Link |
- Amino Acid Metabolism
THF is a cofactor for enzymes that metabolize histidine, serine, glycine, and methionine1. The following link shows that THF is a cofactor for serine hydroxymethyltransferase, the enzyme that converts serine to glycine.
Web Link |
References & Links
- Gropper SS, Smith JL, Groff JL. (2008) Advanced nutrition and human metabolism. Belmont, CA: Wadsworth Publishing.
Links
Purine Synthesis – http://themedicalbiochemistrypage.org/images/purinesynthesis.jpg
Serine to Glycine – http://themedicalbiochemistrypage.org/images/glycine-synthesis.jpg
11.12 Folate Deficiency & Toxicity
Folate deficiency is a vitamin deficiency that affects some Americans. The hallmark symptom of folate deficiency is megaloblastic (aka macrocytic) anemia. Megaloblastic anemia, as the name suggests, is characterized by large, nucleated (most red blood cells do not have a nucleus), immature red blood cells. This occurs because folate is needed for DNA synthesis; without it red blood cells are not able to divide properly1. As a result, fewer and poorer functioning red blood cells are produced that cannot carry oxygen as efficiently as normal red blood cells2.
A maternal folate deficiency can lead to neural tube defects in infants. The exact cause of neural tube defects is unknown, but folate supplementation has been shown to decrease the incidence of neural tube defects3. The most common of these neural tube defects is spina bifida (1 out of 2500 babies born in the United States), which is a failure of the neural tube to close and the spinal cord and its fluid protrude out the infant’s back, as shown below4,5.
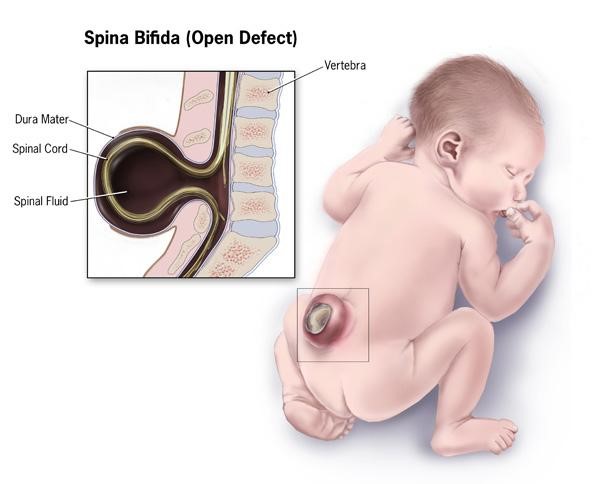
The neural tube closes 21-28 days after conception1, and with 50% of pregnancies estimated to be unplanned, many women aren’t aware they are pregnant during this period1,2. Thus, it is recommended that women of childbearing age consume 400 ug of folic acid daily1. In addition, in 1998 the FDA mandated that all refined cereals and grains be fortified with 140 ug folic acid /100 grams of product7. As you can see below, spina bifida prevalence rates declined during the optional fortification years and declined further once fortification became mandatory in the United States.
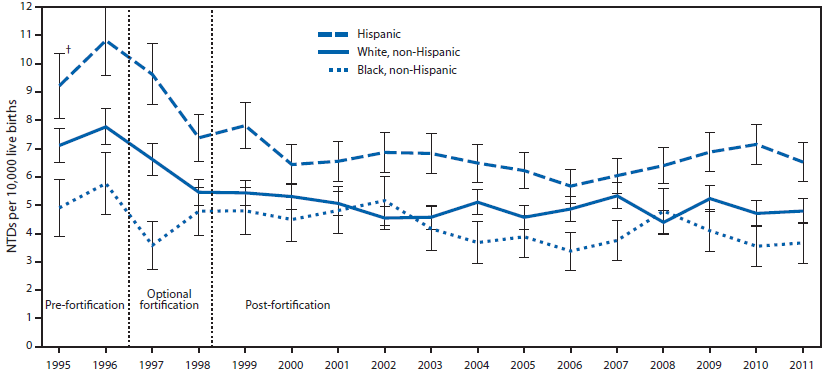
However, more recent research has found that folic acid supplementation begun before conception reduced the occurrence and severity of neural tube defects9.
The following link is an interesting account of the history that led up to the folic acid fortification. It is debatable whether folic acid fortification was fully responsible for the decrease in spina bifida rates shown above, but the rates are lower than they were pre-fortification. The second link is to the announcement that in 2016 the FDA approved the fortification of corn masa flour.
Web Link
Folic Acid Fortification: Fact and Folly (inactive link as of 05/18/2021) |
Folate/Folic acid is not toxic, but it can mask a vitamin B12 deficiency and prevent its diagnosis. This effect will be discussed further in the vitamin B12 deficiency section.
References & Links
- Byrd-Bredbenner C, Moe G, Beshgetoor D, Berning J. (2009) Wardlaw’s perspectives in nutrition. New York, NY: McGraw-Hill.
- Whitney E, Rolfes SR. (2008) Understanding nutrition. Belmont, CA: Thomson Wadsworth.
- Stipanuk MH. (2006) Biochemical, physiological, & molecular aspects of human nutrition. St. Louis, MO: Saunders Elsevier.
- http://www.cdc.gov/ncbddd/birthdefects/SpinaBifida.htm
- http://www.nlm.nih.gov/medlineplus/ency/imagepages/19087.htm
- https://www.cdc.gov/ncbddd/spinabifida/facts.html
- Gropper SS, Smith JL, Groff JL. (2008) Advanced nutrition and human metabolism. Belmont, CA: Wadsworth Publishing.
- http://www.cdc.gov/mmwr/preview/mmwrhtml/mm6401a2.htm
- Bergman JEH, Otten E, Verheij JBGM, de Walle HEK. (2016) Folic acid supplementation influences the distribution of neural tube defect subtypes: A registry-based study. Reprod Toxicol. 59:96-100.
Link
Folic Acid Fortification: Fact and Folly – http://www.fda.gov/AboutFDA/WhatWeDo/History/ProductRegulation/SelectionsFromFDLIUpdateseriesonFDAHistory/ucm091883.htm
FDA Approves Folic Acid Fortification of Corn Masa Flour – https://www.fda.gov/NewsEvents/Newsroom/PressAnnouncements/ucm496104.htm
11.2 Vitamin B12
Vitamin B12 is unique among vitamins in that it contains an element (cobalt) and is found almost exclusively in animal products. Neither plants nor animals can synthesize vitamin B12. Instead, vitamin B12 in animal products is produced by microorganisms within the animal that the products came from. Animals consume the microorganisms in soil or bacteria in ruminant animals that produce vitamin B121. Some plant products, such as fermented soy products (tempeh, miso) and the sea algae supplement, spirulina, are advertised as being good sources of B12. However, fermented soy products are not a reliable vitamin B12 source2 and spirulina contains a pseudovitamin B12 compound that is not bioavailable3. For vegans, supplements, nutritional yeast, and fortified products like fortified soy milk can help them meet their vitamin B12 needs4.
Vitamin B12’s scientific name is cobalamin, which makes sense when you consider it contains cobalt and many amine groups, as shown in the figure below.
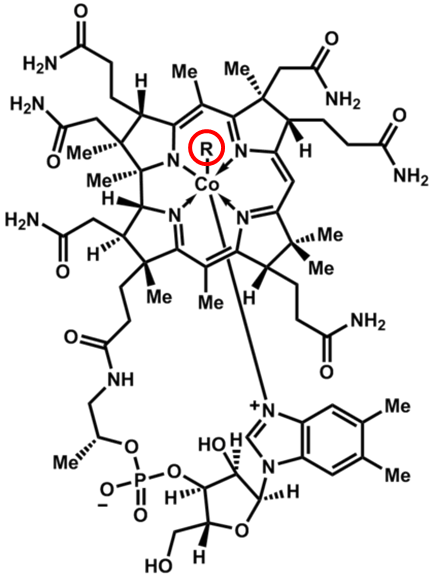
The other feature that is important in cobalamin is the circled R group. This is what differs between the different cobalamins, whose names and R groups are shown in the following table.
Table 11.21 Different cobalamin forms
R Group | Name |
CN | Cyanocobalamin |
OH | Hydroxocobalamin |
H2O | Aquocobalamin |
NO2 | Nitritocobalamin |
5′-deoxyadenosyl | Adenosylcobalamin* |
CH3 | Methylcobalamin* |
*Cofactor Forms
The 2 cofactor forms are adenosylcobalamin and methylcobalamin. We can convert most cobalamins into these 2 cofactor forms. Most foods contain adenosylcobalamin, hydroxocobalamin, or methylcobalamin6. The most common form found in supplements is cyanocobalamin, with some also using methylcobalamin7. Cyanocobalamin is a synthetic form of the vitamin B12.
The uptake, absorption, and transport of vitamin B12 is a complex process. The following descriptions explain, and figures illustrate, this process.
Vitamin B12 is normally bound to protein in food. Salivary glands in the mouth produce haptocorrin (formerly known as R protein), which travels with the food into the stomach. In the stomach, acid converts pepsinogen into pepsin, and the protein intrinsic factor is released from the parietal cells1,8.
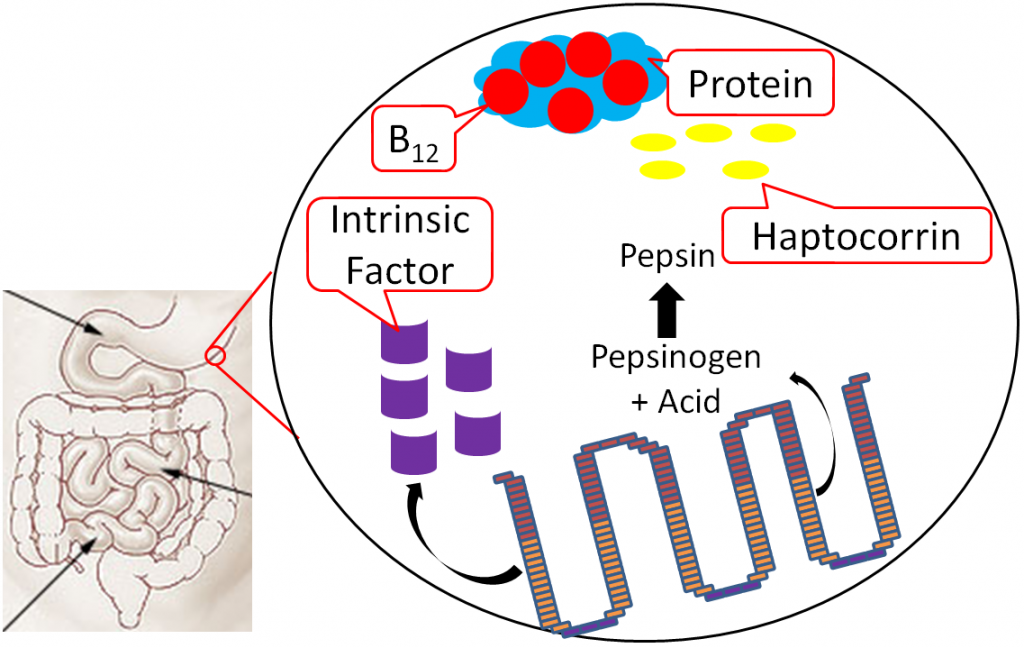
As pepsin frees B12 from protein, haptocorrin binds to the newly freed vitamin B12 (haptocorrin + B12). Intrinsic factor escapes digestion and, along with haptocorrin + B12, exits the stomach and enters the duodenum1,8.
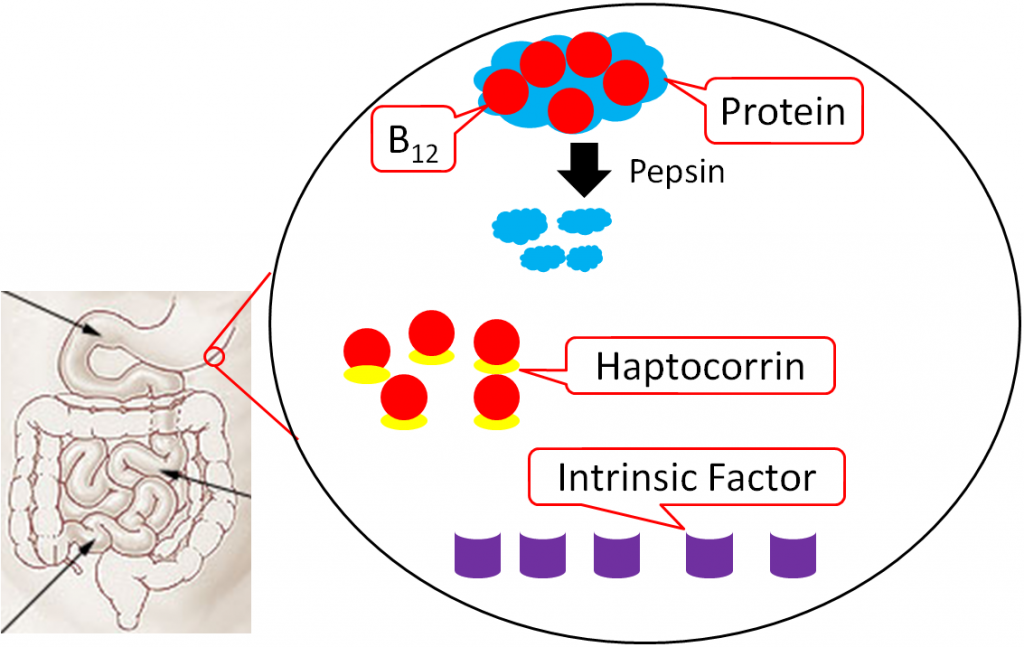
In the duodenum, pancreatic proteases break down haptocorrin, and again vitamin B12 is freed. Intrinsic factor then binds vitamin B12 (intrinsic factor + B12); intrinsic factor + B12 continues into the ileum to prepare for absorption1,8.
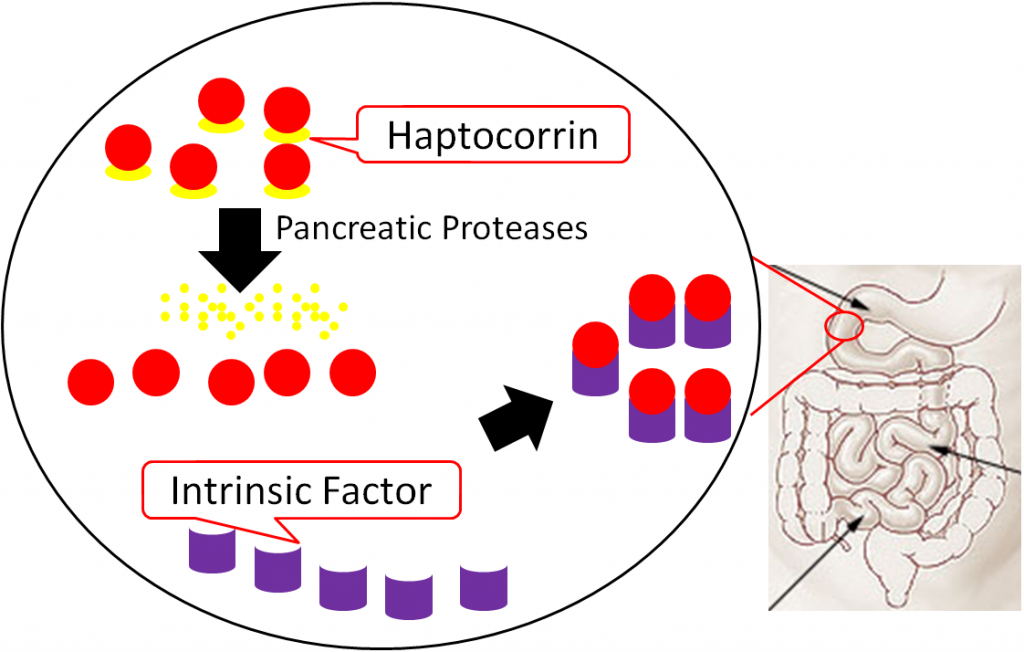
In the ileum, intrinsic factor + B12 is believed to be endocytosed by cubulin (aka intrinsic factor receptor), forming an endosome inside the enterocyte. Intrinsic factor is broken down in the enterocyte, freeing vitamin B12. The free vitamin B12 is then bound to transcobalamin II (TC II + B12); TC II + B12 moves into circulation8.
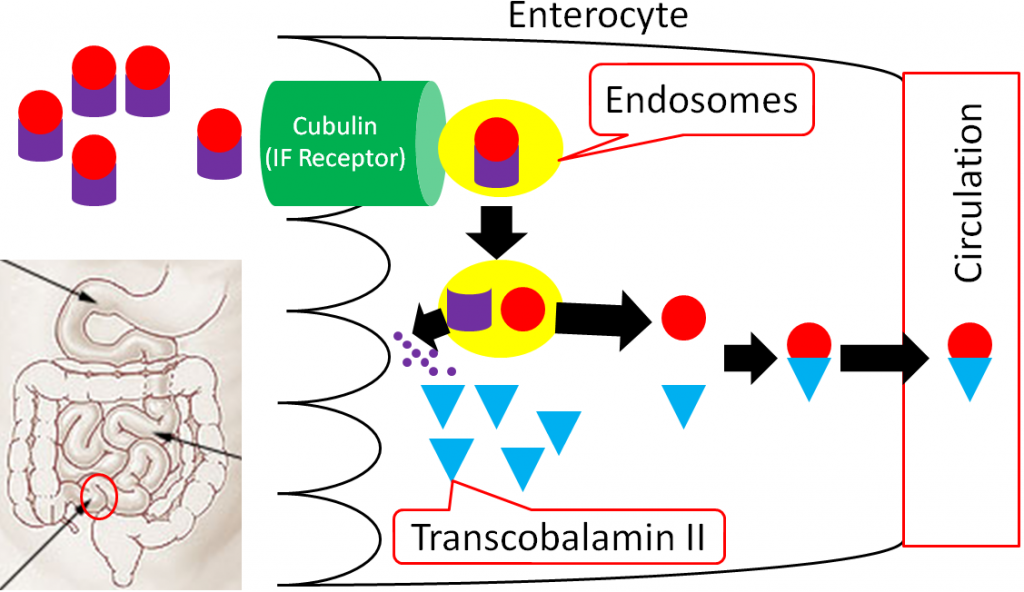
The liver is the primary storage site for vitamin B12. Unlike most other water-soluble vitamins, the liver is able to maintain significant stores of vitamin B12. Uptake into the liver occurs through the binding of TC II + B12 to the TC II Receptor and the endocytosis of both the compound and the receptor8. Vitamin B12 is once again freed after degradation of TC II. Vitamin B12 is primarily stored in the liver as adenosylcobalamin6,8.
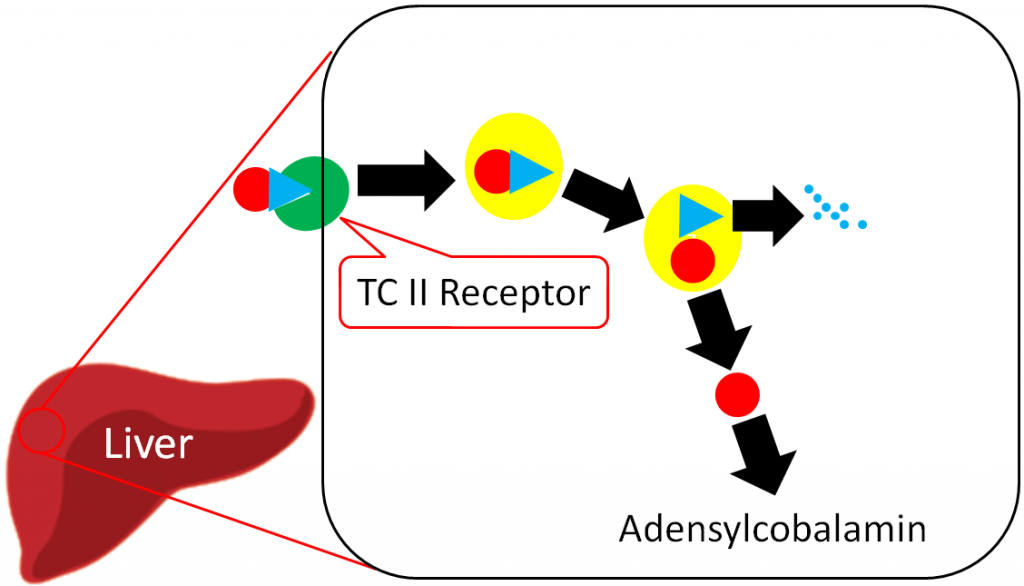
The overall bioavailability of vitamin B12 is believed to be approximately 50%3, with the different cobalamin forms having similar bioavailabilities7. Sublingual supplements of vitamin B12 have been found to be equally efficacious as oral supplements7. Excretion occurs mostly through bile, with little loss in urine6.
Subsections:
11.21 Vitamin B12 Functions
11.22 Vitamin B12 Deficiency & Toxicity
References & Links
- Byrd-Bredbenner C, Moe G, Beshgetoor D, Berning J. (2009) Wardlaw’s perspectives in nutrition. New York, NY: McGraw-Hill.
- Craig W, Mangels A. (2009) Position of the american dietetic association: Vegetarian diets. J Am Diet Assoc 109(7): 1266-1282.
- Watanabe F. (2007) Vitamin B12 sources and bioavailability. Exp Biol Med 232(10): 1266-1274.
- http://www.vrg.org/nutrition/b12.php
- http://en.wikipedia.org/wiki/File:Cobalamin.png
- Gropper SS, Smith JL, Groff JL. (2008) Advanced nutrition and human metabolism. Belmont, CA: Wadsworth Publishing.
- https://ods.od.nih.gov/factsheets/VitaminB12-HealthProfessional/
- Shils ME, Shike M, Ross AC, Caballero B, Cousins RJ, editors. (2006) Modern nutrition in health and disease. Baltimore, MD: Lippincott Williams & Wilkins.
- http://commons.wikimedia.org/wiki/File:Illu_small_intestine_catal%C3%A0.png
11.21 Vitamin B12 Functions
Vitamin B12 is a cofactor for 2 enzymes:
- Methionine synthase
- Methylmalonyl mutase
Methionine Synthase
Methionine synthase is an important enzyme in 1-carbon metabolism that uses methylcobalamin as its cofactor and converts homocysteine to methionine by adding a methyl group. Methionine then is converted to other compounds that serve as methyl donors, as shown below1.
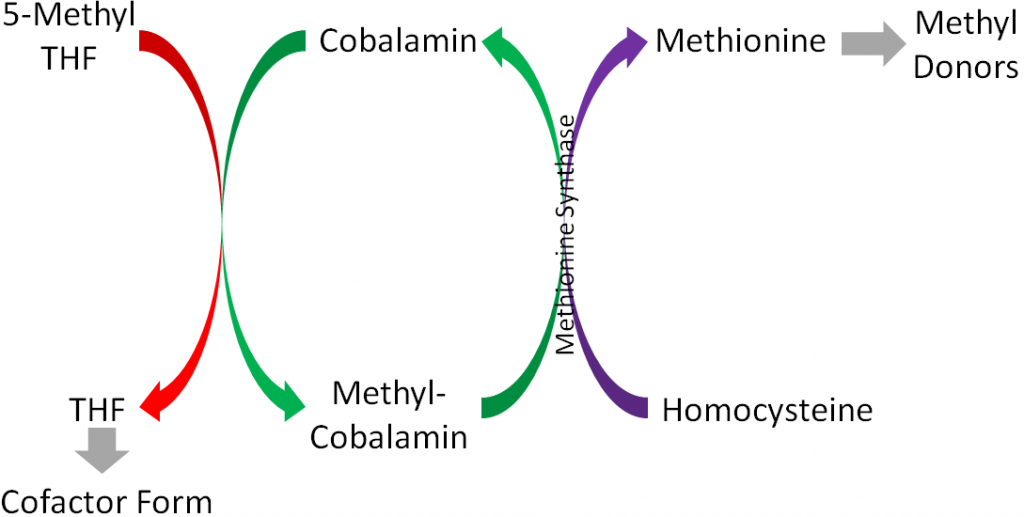
These methyl donors can donate methyl groups for methylating DNA, an epigenetic modification1.
Methymalonyl mutase
This enzyme uses adenosylcobalamin as its cofactor, and is important in the breakdown of odd chain fatty acids (5 carbons etc.). As you know, odd chain fatty acids are less common than even chain fatty acids, but this enzyme is required to properly handle these less common fatty acids1.
Demyelination
In addition to its role as a cofactor for enzymes, vitamin B12 is also important for preventing degradation of the myelin sheath that surrounds neurons, as shown below.
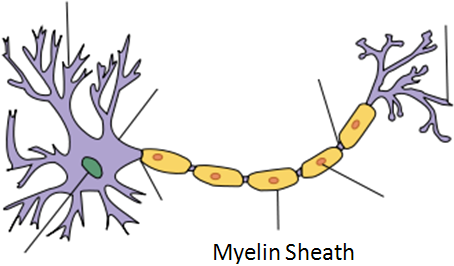
The mechanism through which vitamin B12 prevents demyelination is not known3.
References & Links
- Byrd-Bredbenner C, Moe G, Beshgetoor D, Berning J. (2009) Wardlaw’s perspectives in nutrition. New York, NY: McGraw-Hill.
- https://en.wikipedia.org/wiki/Myelin#/media/File:Neuron_Hand-tuned.svg
- http://lpi.oregonstate.edu/infocenter/vitamins/vitaminB12/
11.22 Vitamin B12 Deficiency & Toxicity
There are 2 primary symptoms of vitamin B12 deficiency:
Megaloblastic (Macrocytic) Anemia
Neurological Abnormalities
Megaloblastic (Macrocytic) Anemia
This is the same type of anemia that occurs in folate deficiency that is characterized by fewer, enlarged, immature red blood cells. In vitamin B12 deficiency, this can occur because there is not enough cobalamin to convert 5-methyl THF to THF (illustrated in Figure 11.211). Thus, THF is not available for normal DNA synthesis and the red blood cells do not divide correctly.
Neurological Abnormalities
Vitamin B12 deficiency also results in nerve degeneration and abnormalities that can often precede the development of anemia. These include a decline in mental function and burning, tingling, and numbness of legs. These symptoms can continue to worsen and deficiency can be fatal1.
The most common cause of vitamin B12 deficiency is pernicious anemia, a condition of inadequate intrinsic factor production that causes poor vitamin B12 absorption. This condition is common in people over the age of 50 because they have the condition atrophic gastritis2. Atrophic gastritis is a chronic inflammatory condition that leads to the loss of glands in the stomach, as shown in the figure in the following link.
Web Link |
The loss of glands leads to decreased intrinsic factor production. It is estimated that ~6% of those age 60 and over are vitamin B12 deficient, with 20% having marginal status3. In addition to the elderly, vegans are also at risk for vitamin B12 deficiency because they do not consume animal products. However, the deficiency may take years to develop in adults because of stores and recycling of vitamin B122. Deficiency has the potential to occur much quicker in infants or young children on vegan diets because they do not have stores that adults do4.
Folate/Folic Acid masking vitamin B12 deficiency
As mentioned above, folate and vitamin B12 lead to the same megaloblastic (macrocytic) anemia. If high levels of folate or folic acid (most of the concern is with folic acid since it is fortified in foods and commonly taken in supplements) is given during vitamin B12 deficiency, it can correct this anemia. This is referred to as masking because it does not rectify the deficiency, but it “cures” this symptom. This is problematic because it does not correct the more serious neurological problems that can result from vitamin B12 deficiency. There are some people who are concerned about the fortification of cereals and grains with folic acid because people who are B12 deficient might not develop macrocytic anemia, which makes a vitamin B12 deficiency harder to diagnose2.
No toxicity of vitamin B12 has been reported.
References & Links
- Byrd-Bredbenner C, Moe G, Beshgetoor D, Berning J. (2009) Wardlaw’s perspectives in nutrition. New York, NY: McGraw-Hill.
- Whitney E, Rolfes SR. (2008) Understanding nutrition. Belmont, CA: Thomson Wadsworth.
- Allen L. (2009) How common is vitamin B-12 deficiency? Am J Clin Nutr 89(2): 693S-696S.
- Gropper SS, Smith JL, Groff JL. (2008) Advanced nutrition and human metabolism. Belmont, CA: Wadsworth Publishing.
Links
Atrophic Gastritis – http://book-med.info/gastritis/12812
11.3 B Vitamins, Homocysteine & Cardiovascular Disease
Homocysteine is a sulfur containing, non-proteinogenic (not used for making proteins) amino acid whose structure is shown in the figure below.
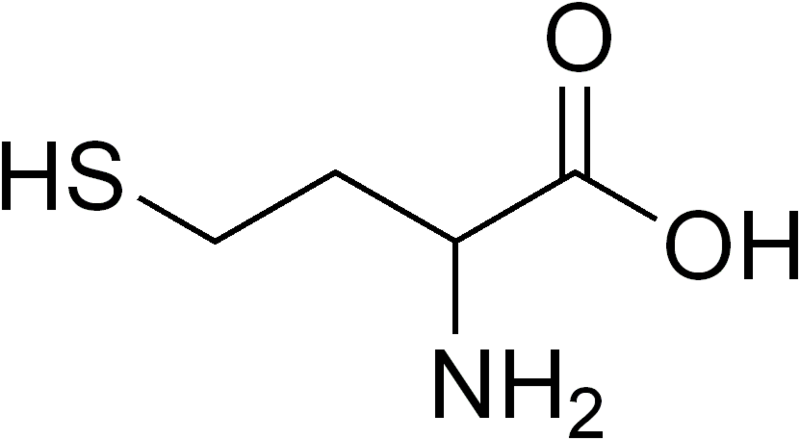
Elevated circulating homocysteine levels have been found in people with cardiovascular disease. Folate, vitamin B6, and vitamin B12 contribute to the conversion of homocysteine to methionine by providing methyl groups, thereby decreasing homocysteine levels, as illustrated in the figure below. Thus, based on these facts, it was hypothesized that intake of these B vitamins may decrease the risk of cardiovascular disease.
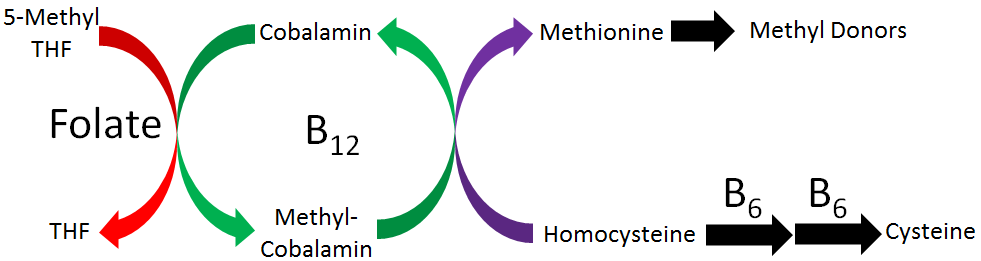
Research has found that intake of these B vitamins does decrease circulating homocysteine levels. However, most studies have not found that it results in improved cardiovascular disease outcomes2-4. It is debated why B vitamin intake has not resulted in improved outcomes. Some think it is because the studies have not focused on individuals with elevated homocysteine levels2, while others believe that homocysteine is a biomarker or indicator of cardiovascular disease, not a causative or contributing factor to cardiovascular disease development3.
References & Links
- http://en.wikipedia.org/wiki/File:Homocysteine_racemic.png
- Abraham J, Cho L. (2010) The homocysteine hypothesis: Still relevant to the prevention and treatment of cardiovascular disease? Cleve Clin J Med 77(12): 911-918.
- Cacciapuoti F. (2011) Hyper-homocysteinemia: A novel risk factor or a powerful marker for cardiovascular diseases? pathogenetic and therapeutical uncertainties. J Thromb Thrombolysis 32(1): 82-88.
- Martai-Carvajal AJ, Sola J, Lathyris D. (2015) Homocysteine-lowering interventions for preventing cardiovascular events. Cochrane Database Syst Rev. 1:CD006612.