Main Body
Antioxidant Micronutrients
This chapter will describe what antioxidants are and then discuss the three major antioxidant micronutrients: vitamin E, vitamin C and selenium.
9.1 Antioxidants
9.2 Vitamin E
9.3 Vitamin C
9.4 Selenium
9.1 Antioxidants
The antioxidant vitamins and minerals are:
Vitamin E
Vitamin C
Selenium
Iron
Copper
Zinc
Manganese
Riboflavin
In this section, we are going to cover vitamin E, vitamin C, and selenium in detail because being an antioxidant is their primary function. Iron, copper, zinc, and manganese are cofactors for the antioxidant enzymes catalase and superoxide dismutase, as shown below.
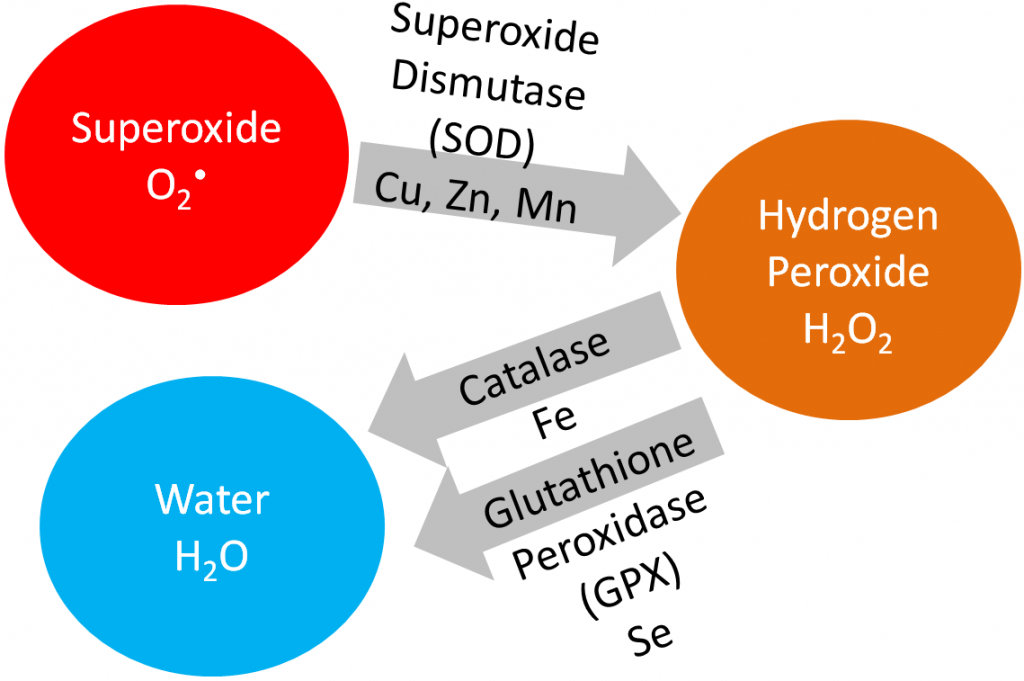
Superoxide dismutase converts superoxide into hydrogen peroxide. Catalase converts hydrogen peroxide into water. Iron, copper, and zinc will be covered in more detail in the blood, bones, and teeth chapter (chapter 11). Manganese will be covered in the macronutrient metabolism chapter.
Riboflavin, in the cofactor FAD, is an important cofactor for several antioxidant enzymes, but it will be covered in more depth in the macronutrient metabolism micronutrients chapter (Chapter 10).
Subsections:
9.11 Free Radicals & Oxidative Stress
9.12 What is an Antioxidant?
9.13 Meaningful Antioxidant(s)
9.14 Too Much of a Good Thing? Antioxidants as Pro-oxidants
9.11 Free Radicals & Oxidative Stress
Before you can understand what an antioxidant is, it is important to have an understanding of oxidants. As you have learned already, oxidation is the loss of an electron as shown below.
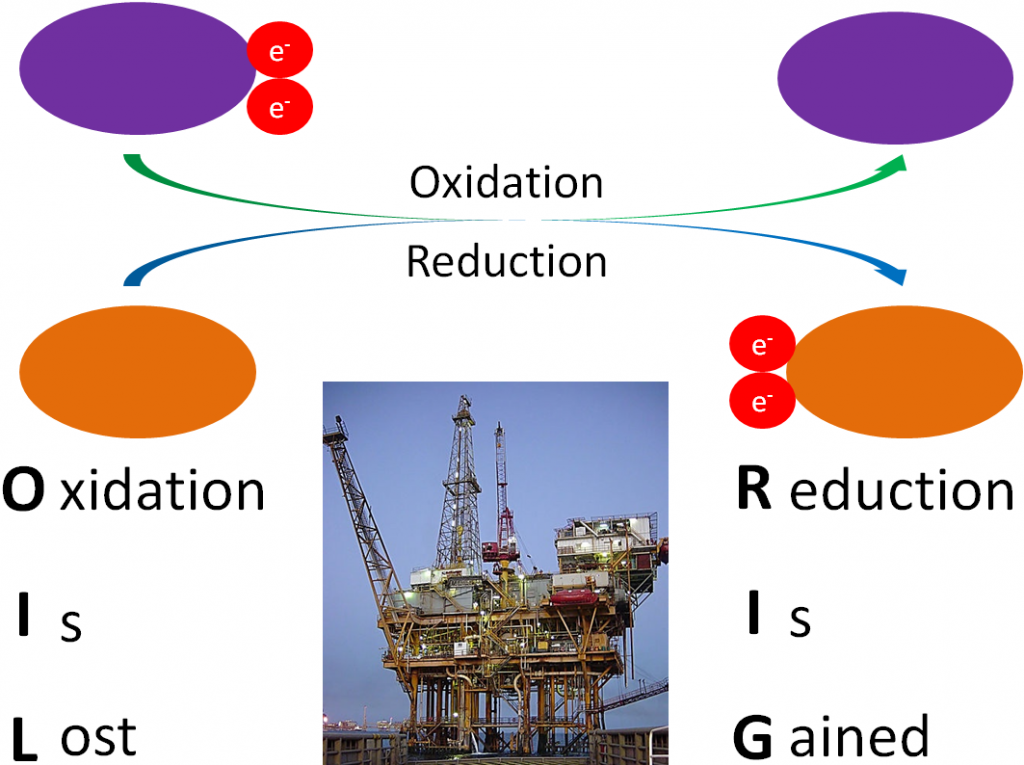
Some important terms to understand:
Free Radical – a molecule with an unpaired electron in its outer orbital.
The following example shows normal oxygen losing an electron from its outer orbital and thus, becoming an oxygen free radical.
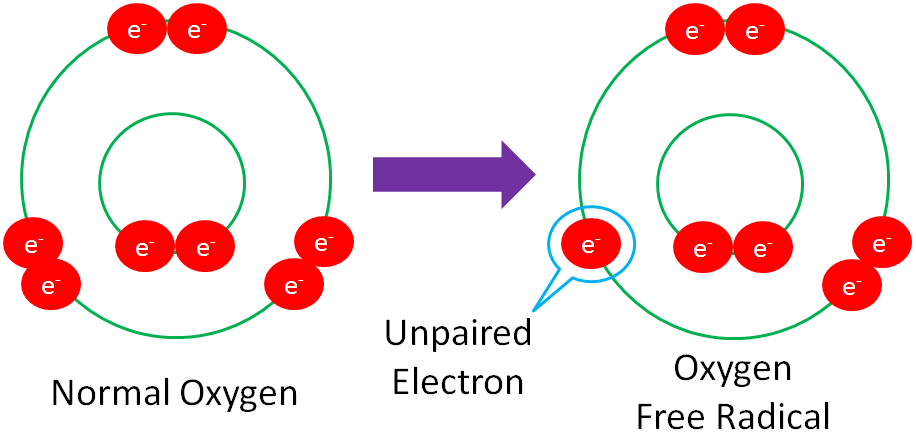
Free radicals are highly reactive because they actively seek an electron to stabilize the molecule.
Reactive Oxygen Species (ROS) – an oxygen-containing, free radical species.
Some of the most common ROS are (● symbolizes radical):
Superoxide (O2●)
Hydroxyl Radical (●OH)
Hydrogen Peroxide Radical (HO2●)
Peroxyl Radical (ROO2●)
Alkoxyl Radical (RO●)
Ozone (O3)
Singlet Oxygen (1O2)
Hydrogen Peroxide (H2O2)
Oxidative Stress – the imbalance between the production of ROS/free radicals and the body’s ability to quench them.
The following video does a good job illustrating how free radicals can be formed and quenched by antioxidants.
Web Link |
The following figure shows that inflammation caused by hitting your thumb with a hammer, exposure to UV light, radiation, smoking, and air pollution are all sources of free radicals.
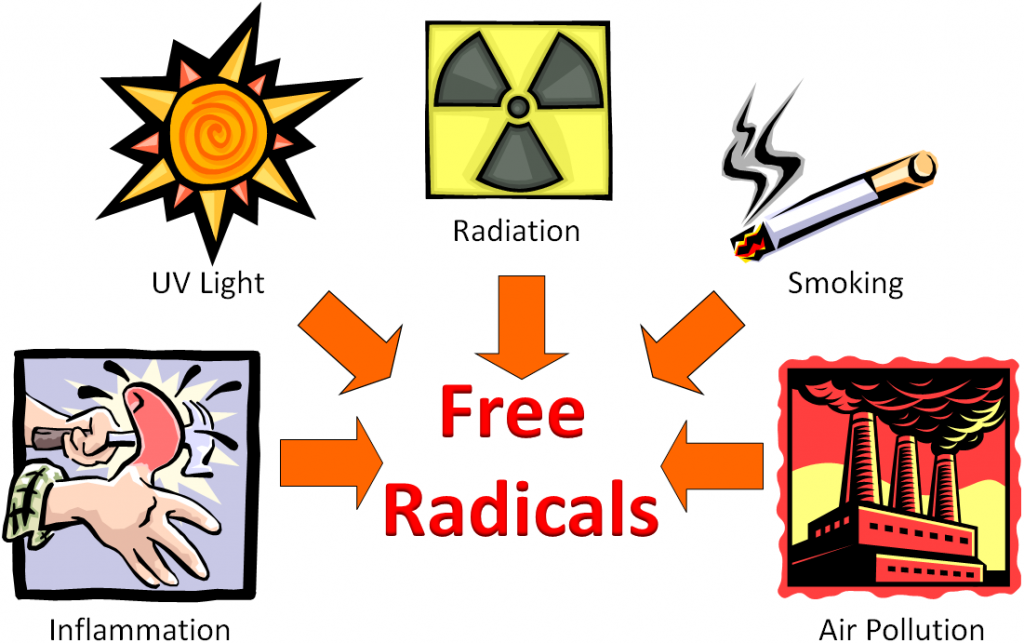
Free radicals can be generated by a variety of sources that can be classified as endogenous (within the body) and exogenous sources (outside the body).
So, we have these free radicals searching for an electron, what’s the big deal? The problem arises if the free radicals oxidize LDL, proteins, or DNA as shown below.
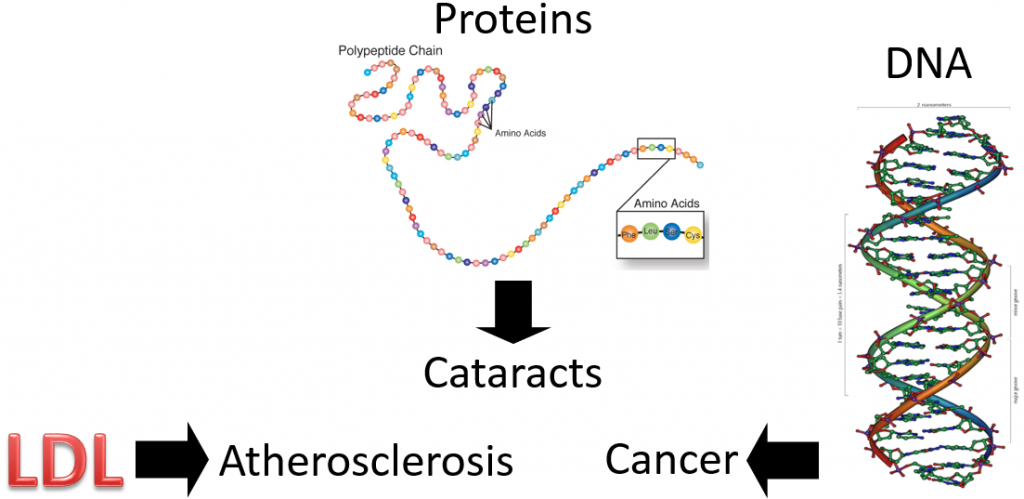
Oxidized LDL is more atherogenic, meaning it is more likely to contribute to atherosclerosis (hardening of the arteries) than normal LDL. Protein oxidation is believed to be involved in the development of cataracts. Cataracts are the clouding of the lens of the eye. If you would like to see what it looks like, see the link below.
Web Link |
If a nucleotide in DNA is attacked, it can result in a mutation. A mutation is a change in the nucleotide or base pair sequence of DNA. Mutations are a common occurrence in cancer.
References & Links
- http://en.wikipedia.org/wiki/Image:Gulf_Offshore_Platform.jpg
- http://www.genome.gov/Pages/Hyperion/DIR/VIP/Glossary/Illustration/amino_acid.cfm?key=amino%20acids
- http://www.genome.gov/Glossary/index.cfm?id=149
Videos
How Antioxidants Work – https://www.youtube.com/watch?v=lG3OOXIXvxw
Links
Cataract Vision Simulator – https://www.aao.org/eye-health/diseases/cataracts-vision-simulator
9.12 What is an Antioxidant?
We are ready to move on to antioxidants, which as their name indicates, combat free radicals, ROS, and oxidative stress. As a humorous introduction, the link below is to a cartoon that shows Auntie Oxidant kicking free radicals out of the bloodstream.
Web Link |
But it’s not quite that simple. You have probably heard the saying “take one for the team.” Instead of taking one for the team, antioxidants “give one for the team.” The ‘giving’ is the donation of an electron from the antioxidant to a free radical, in order to regenerate a stable compound, as shown below.
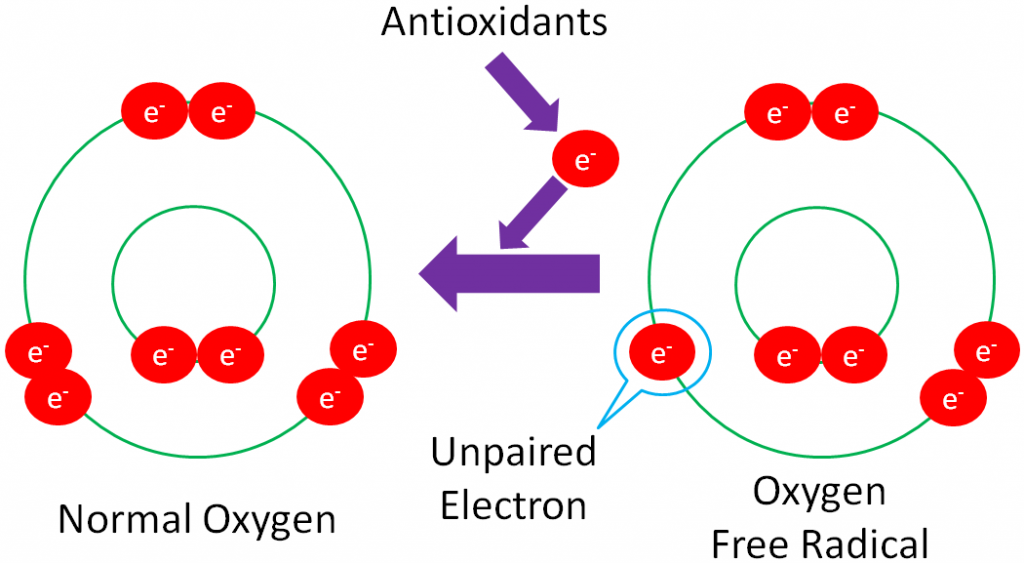
Donating an electron is how vitamins act as antioxidants. Minerals, on the other hand, are not antioxidants themselves. Instead, they are cofactors for antioxidant enzymes.
These antioxidant enzymes include:
Superoxide dismutase (SOD): uses copper, zinc, and manganese as cofactors (there is more than one SOD enzyme); converts superoxide to hydrogen peroxide and oxygen1.
Catalase: uses iron as a cofactor; converts hydrogen peroxide to water1.
Glutathione peroxidase (GPX): is a selenoenzyme that converts hydrogen peroxide to water. It can also convert other reactive oxygen species (ROSs) to water1.
The action of these enzymes is shown below.
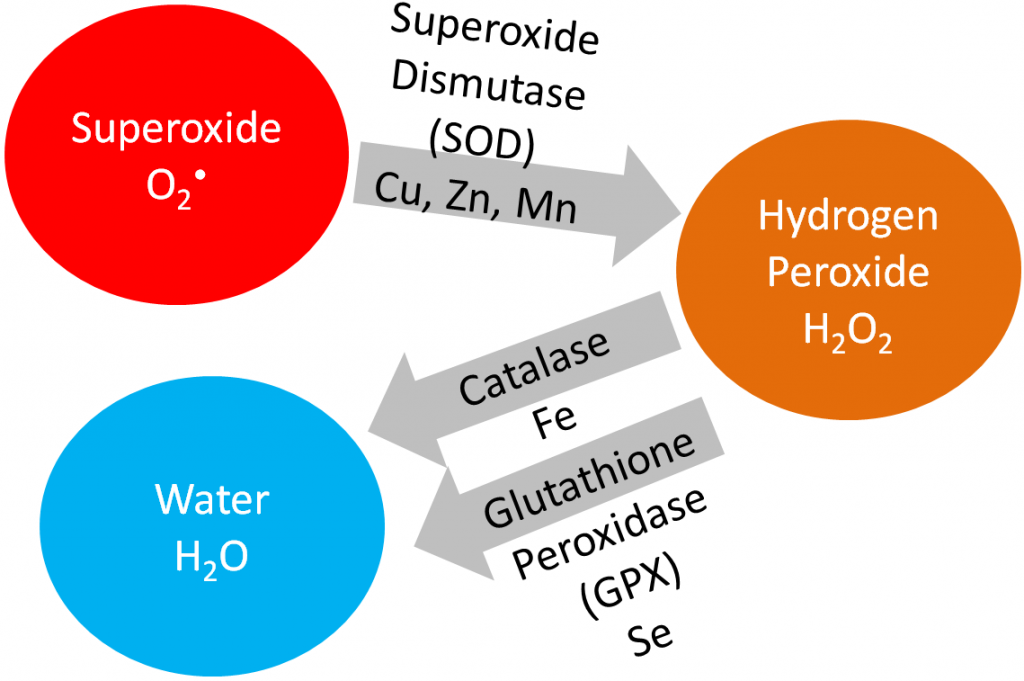
Antioxidants are thought to work in concert with one another, forming what is known as the antioxidant network. An example of the antioxidant network is shown below. Alpha-tocopherol (major form of vitamin E in our body) is oxidized, forming an alpha-tocopherol radical. This donation of an electron stabilizes reactive oxygen species. Ascorbate (vitamin C) is then oxidized, forming dehydroascorbate to regenerate (reduce) alpha-tocopherol. Ascorbate is then regenerated by the selenoenzyme thioredoxin reductase. This demonstrates how antioxidants can function as a network to regenerate one another so they can continue to function as antioxidants.
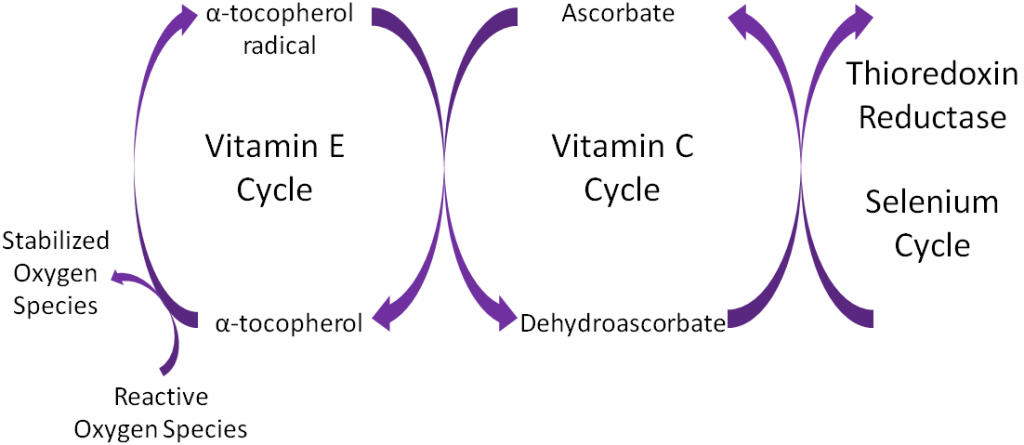
References & Links
- Gropper SS, Smith JL, Groff JL. (2008) Advanced nutrition and human metabolism. Belmont, CA: Wadsworth Publishing.
- Packer L, Weber SU, Rimbach G. (2001) Molecular aspects of alpha-tocotrienol antioxidant action and cell signalling. J Nutr 131(2): 369S-373S.
Link
Auntie Oxidant – http://www.ibiblio.org/Dave/Dr-Fun/df200005/df20000523.jpg (inactive link as of 05/18/2021)
9.13 Meaningful Antioxidant(s)
There is a lot of confusion among the public on antioxidants. For the most part, this is for a good reason. Many food companies put antioxidant numbers on the packages that sound good to consumers, who have no idea how to interpret them. Thus, it is increasingly important to have an understanding of what a meaningful antioxidant actually is.
A meaningful antioxidant has two characteristics (these are based on the assumption that the compound is an antioxidant):
- Found in appreciable amounts right location where there are free radicals/ROS that need to be quenched
- It is not redundant with another antioxidant that is already serving as an antioxidant
What do these mean? Let’s consider the example of lycopene and vitamin E (alpha-tocopherol), which are both fat-soluble antioxidants. In vitro antioxidant assays have found that lycopene is 10-fold more effective in quenching singlet oxygen than alpha-tocopherol1. However, when you look at the concentrations found in the body, there is far more alpha-tocopherol than lycopene. For example:
LDL on average contains 11.6 molecules of alpha tocopherol and 0.9 molecules of lycopene. Thus, if we divide alpha tocopherol by lycopene 11.6/0.9 we find that there is on average 12.9 times more alpha-tocopherol than lycopene1.
Other examples in the body:
Prostate – 162-fold higher alpha-tocopherol than lycopene concentrations
Skin – 17 to 269-fold higher alpha-tocopherol than lycopene concentrations
Plasma – 53-fold higher alpha tocopherol than lycopene concentrations1
Thus, despite the fact that lycopene is a better antioxidant in vitro, since the concentration of alpha-tocopherol is so much higher in tissues (locations of need), it is likely the more meaningful antioxidant. In addition, if lycopene and alpha-tocopherol have similar antioxidant functions (fat-soluble antioxidants), lycopene’s potential antioxidant action is redundant to alpha-tocopherol’s antioxidant function and thus, also less likely to be a meaningful antioxidant. Indeed, further examination of the literature has not suggested that lycopene can act as an antioxidant in vivo, even though it is a good one in vitro1.
You may be wondering “What about the in vitro antioxidant assays, like the oxygen radical absorbance capacity (ORAC) assay that some food and supplement companies are including on their labels?” This is an example of how some companies/businesses use ORAC values to market their product(s). However, the USDA removed its table of ORAC values “due to mounting evidence that the values indicating antioxidant capacity have no relevance to the effects of specific bioactive compounds, including polyphenols on human health2.”
Web Link |
However, going back to the two characteristics of meaningful antioxidants, there really is no evidence that shows that a high ORAC score leads to any benefit in vivo. This is because the measure also doesn’t take into account characteristics such as bioavailability. Bioavailability is the amount of a compound that is absorbed or reaches circulation. Many of these purported super antioxidants have not been shown to be absorbed or maintained in the body in a way that would suggest that they would be meaningful antioxidants. 5 years after it was removed, industry and suppliers think it has been a good thing that it is no longer used as indicated in the following article.
Web Link
Saying goodbye to ORAC was a good thing for industry, suppliers say |
References & Links
- Erdman, J.W., Ford, N.A., Lindshield, B.L. Are the health attributes of lycopene related to its antioxidant function? Arch Biochem Biophys, 483: 229-235, 2009.
- http://www.ars.usda.gov/Services/docs.htm?docid=15866 (inactive link as of 05/18/2021)
Link
Sozo Coffeee ORAC Comparison – http://www.sozoglobal.com/products/?sec=1http://www.sozoglobal.com/products/?sec=1
Saying goodbye to ORAC was a good thing for industry, suppliers say – http://www.nutraingredients-usa.com/Suppliers2/Saying-goodbye-to-ORAC-was-a-good-thing-for-industry-suppliers-say
9.14 Too Much of a Good Thing? Antioxidants as Pro-oxidants
Chapter 1 described a clinical trial that found that high-dose beta-carotene supplementation increased lung cancer risk in smokers. This is an example of findings that support that high doses of antioxidants may be “too much of a good thing”, causing more harm than benefit. The parabolic, or U-shaped figure, below displays how the level of nutrient concentration or intake (x-axis) relates to an antioxidant measure (y-axis). The lowest level of antioxidant intake or tissue concentration results in nutrient deficiency if the antioxidant is essential (vitamins and minerals). Intake levels above deficient, but less than optimal, are referred to as low suboptimal. Suboptimal means the levels are not optimal. Thus, low suboptimal and high suboptimal sandwich optimal. The high suboptimal level is between optimal and where the nutrient becomes toxic.
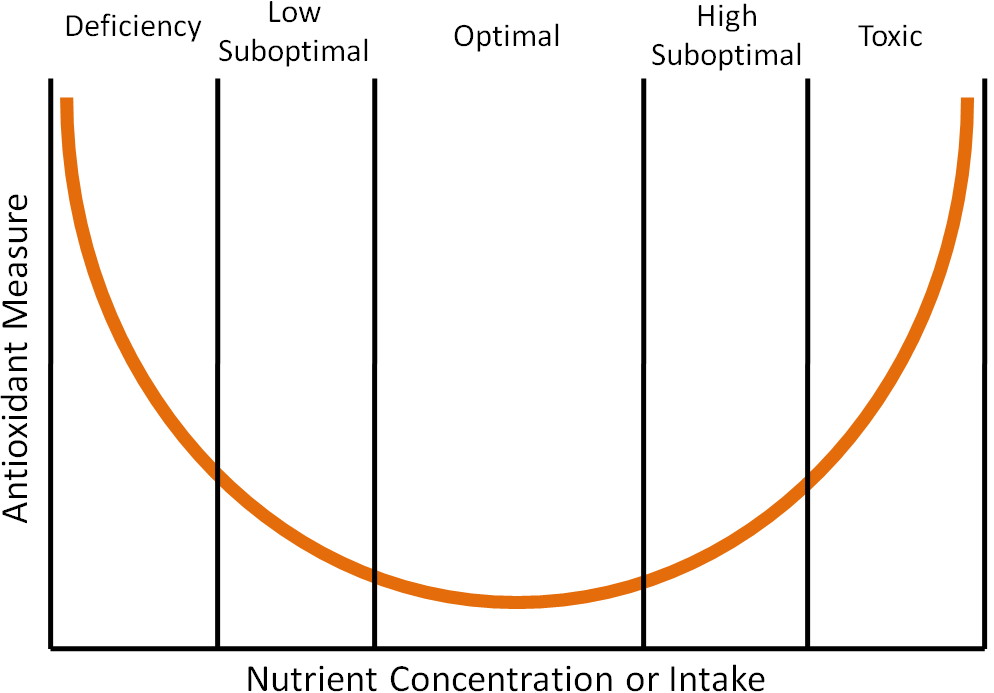
An example of where this phenomenon has been shown to occur is in the dog prostate with toenail selenium concentrations, which are a good indicator of long-term selenium status1. Researchers found that when they plotted prostate DNA damage (antioxidant measure) against toenail selenium status (nutrient concentration or intake) that it resulted in a U-shaped curve like the one shown above1. Thus, it is good to have antioxidants in your diet, but too much can be counterproductive.
References & Links
- Waters DJ, Shen S, Glickman LT, Cooley DM, Bostwick DG, et al. (2005) Prostate cancer risk and DNA damage: Translational significance of selenium supplementation in a canine model. Carcinogenesis 26(7): 1256-1262.
9.2 Vitamin E
There are 8 different forms of vitamin E: 4 tocopherols and 4 tocotrienols. The difference between tocopherols and tocotrienols is that the former have a saturated tail, while the latter have an unsaturated tail. Within tocopherols and tocotrienols, the difference between the different forms is the position of the methyl groups on the ring. The 4 different forms within the tocopherol and tocotrienols are designated by the Greek letters: alpha, beta, gamma, and delta. The difference in these structures is shown in the figures below.
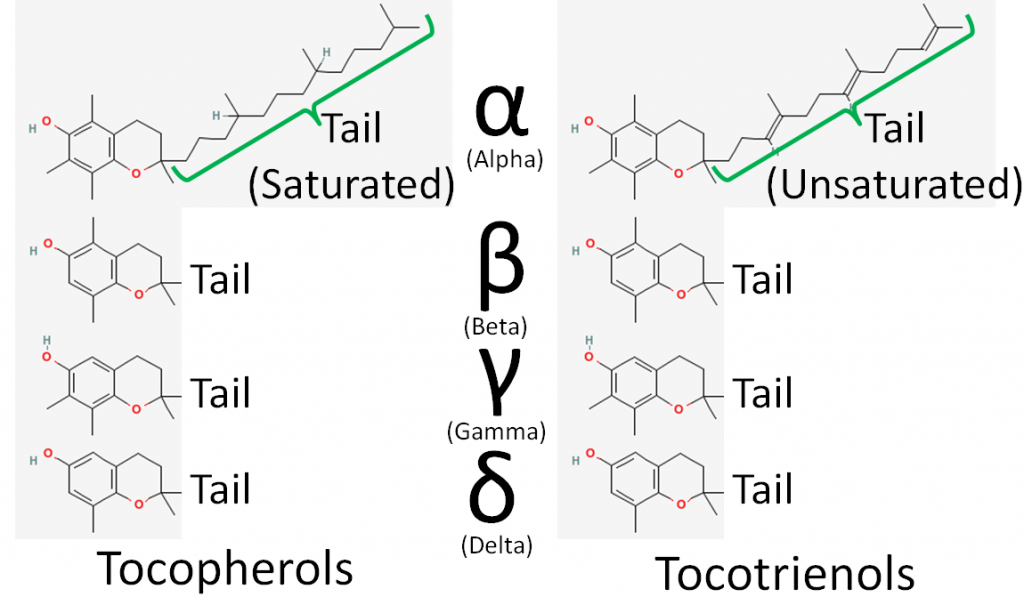
For reasons that will be covered in a later subsection, the primary form of vitamin E found in the body is alpha-tocopherol. The major, and possibly only, function of vitamin E is as an antioxidant. When it serves as an antioxidant it forms an alpha-tocopherol radical, as shown below.
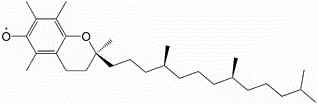
Alpha-tocopherol is believed to be the first part of an antioxidant network (shown below) where it is oxidized to donate an electron to stabilize reactive oxygen species. Alpha-tocopherol radical can then be reduced by the donation of an electron from ascorbate.
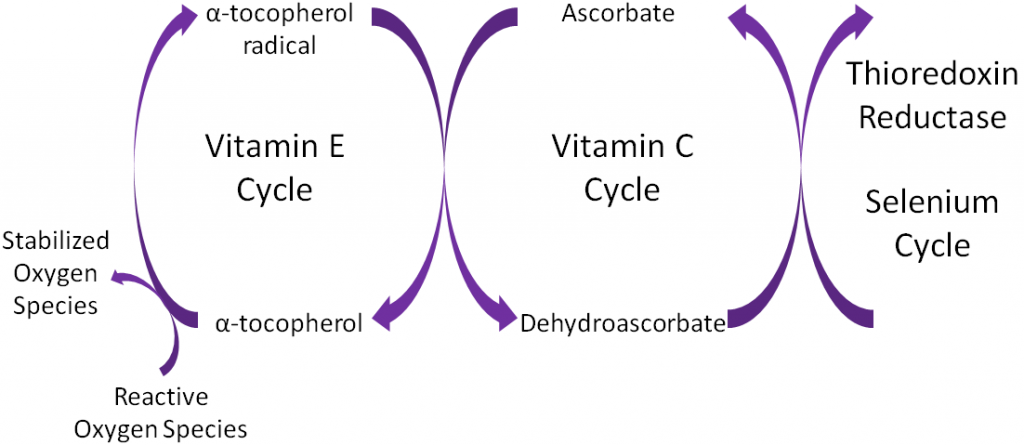
To help protect the antioxidant function of alpha-tocopherol (by preventing the formation of alpha-tocopherol radical) in foods and during digestion, some manufacturers have added compounds to this site of alpha-tocopherol through ester bonds. These are referred to as alpha-tocopherol derivatives or alpha-tocopherol esters. The most common forms are alpha-tocopherol acetate, alpha-tocopherol succinate, and alpha-tocopherol phosphate (Ester-E®). The figures below show the structure of alpha-tocopherol acetate, and the structure of succinic acid.
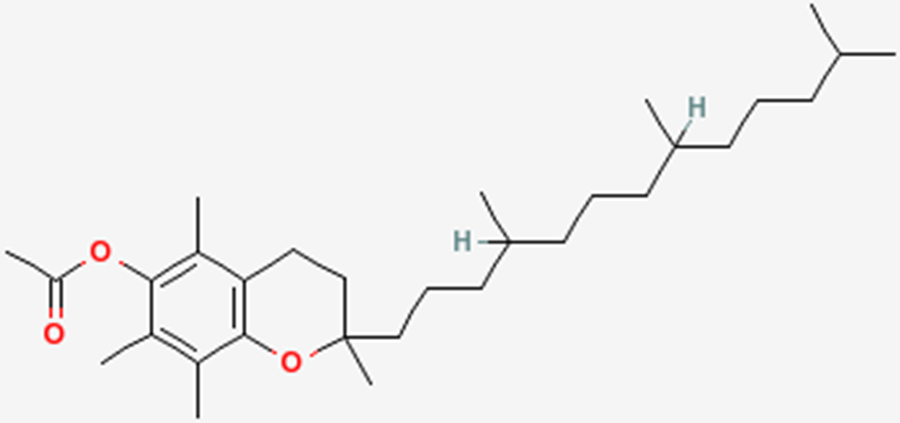
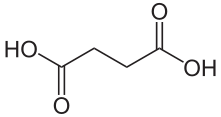
Alpha-tocopherol derivatives, such as acetate in alpha-tocopherol acetate, are cleaved prior to absorption in the small intestine by esterases, meaning that alpha-tocopherol is absorbed, not the alpha-tocopherol derivative.
Subsections:
9.21 Alpha-Tocopherol: Natural vs. Synthetic
9.22 Absorption, Metabolism & Excretion of Vitamin E
9.23 Dietary Vitamin E & Amounts Found in Body
9.24 Vitamin E Deficiency & Toxicity
9.25 Vitamin E DRI & IUs
References & Links
- https://en.wikipedia.org/wiki/Radical_(chemistry)#/media/File:VitE.gif
- Packer L, Weber SU, Rimbach G. (2001) Molecular aspects of alpha-tocotrienol antioxidant action and cell signalling. J Nutr 131(2): 369S-373S
- http://en.wikipedia.org/wiki/File:Bernsteins%C3%A4ure2.svg
9.21 Alpha-Tocopherol: Natural vs. Synthetic
In addition to being found naturally in foods, alpha-tocopherol can also be synthesized. It is important to know whether alpha-tocopherol is natural or synthetic because the stereochemistry differs between these forms. Alpha-tocopherol contains 3 chiral centers (non-superimposable mirror images) designated as R or S. If this is a new topic to you or you would like a refresher, the following link is a fun, animated chirality game.
Web Link
Chirality Game (inactive link as of 05/18/2021) |
The 3 chiral centers in alpha-tocopherol are located at the 2, 4’, and 8’ positions. You can see the full numbering of tocopherols in the link below. In short, the rings are normal numbers and the tail are prime numbers.
Web Link |
The figure below shows the 3 chiral centers without the other numbers.
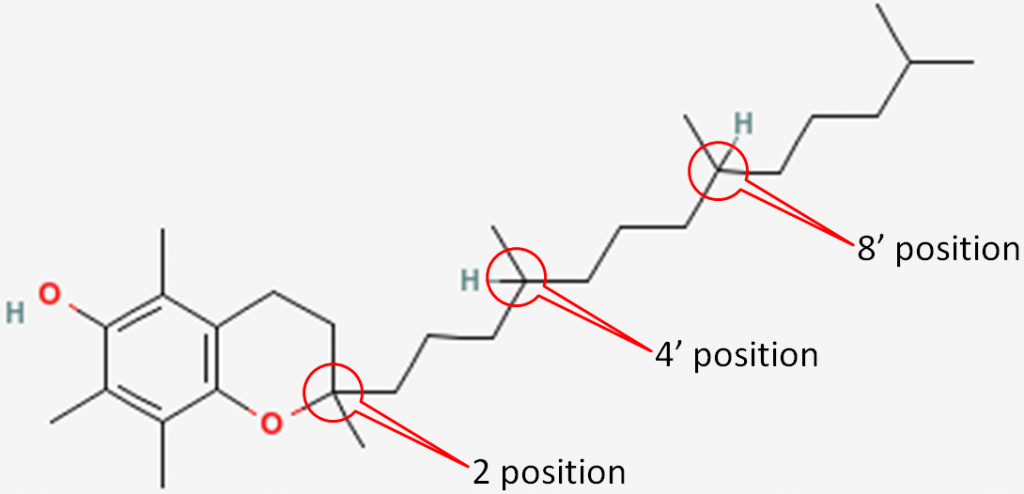
In natural alpha-tocopherol, all 3 chiral centers are in the R configuration. Thus, it is designated RRR-alpha-tocopherol. The R’s represent the 2, 4’, and 8’ positions of alpha-tocopherol, respectively, as shown below1.
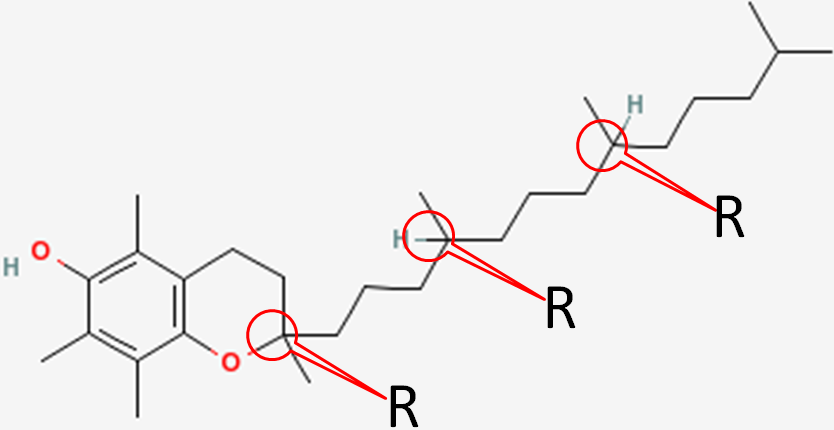
Synthetic alpha-tocopherol is a racemic (equal) mixture of all the different stereochemical possibilities at the three chiral centers. These are:
RRR
RRS
RSS
RSR
SRR
SSR
SSS
SRS
The two forms of alpha-tocopherol are designated (these are placed before alpha-tocopherol to indicate whether it is natural or synthetic) as listed below:
- Natural
New designation: RRR-alpha-tocopherol (because all 3 positions are RRR)
Old designation: d-alpha-tocopherol
- Synthetic
New designation: all-rac-alpha-tocopherol (because it is a racemic mixture)
Old designation: dl-alpha-tocopherol
The old d and dl designations were describing the chemical structure that are sometimes still used. Keep in mind the natural and synthetic are describing the stereochemistry of alpha-tocopherol and not whether it is naturally derived. For example, there are natural alpha-tocopherol derivatives where the derivatives are added through synthetic procedures.
References & Links
- http://lpi.oregonstate.edu/mic/vitamins/vitamin-E
Link
Chirality Game – http://nobelprize.org/educational_games/chemistry/chiral/game/game.html (inactive link as of 05/18/2021)
Tocopherol Numbering – http://www.chem.qmul.ac.uk/iupac/misc/noGreek/toc.html
9.22 Vitamin E Absorption, Metabolism, & Excretion
You might be saying to yourself, “who cares about natural versus synthetic alpha-tocopherol.” But the small change in stereochemistry makes a big difference in how alpha-tocopherol is maintained in the body.
All forms of vitamin E (tocopherols, tocotrienols) are absorbed equally. Fat-soluble vitamins are handled like lipids and thus are incorporated into chylomicrons that have triglycerides removed by lipoprotein lipase. The chylomicron remnants containing the different forms of vitamin E are then taken up by the liver. The figure below shows the absorption, metabolism, and excretion of vitamin E.
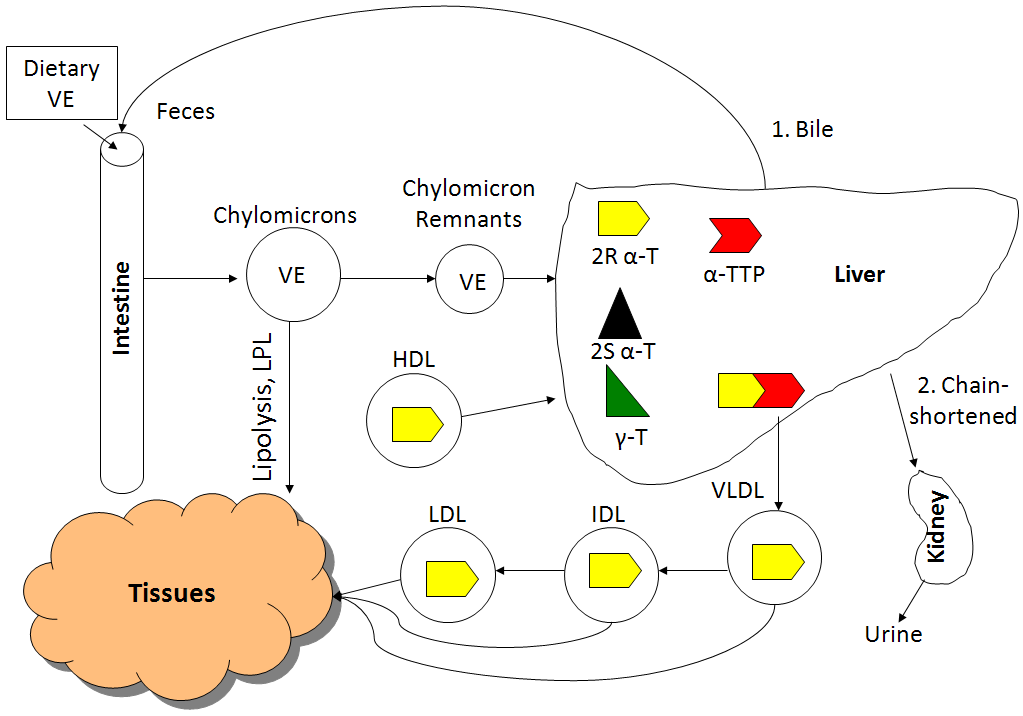
The liver contains a protein called alpha-tocopherol transfer protein (alpha-TTP), which is responsible for maintaining higher levels of alpha-tocopherol in the body. Alpha-TTP preferentially binds to 2R alpha-tocopherol and helps facilitate its incorporation into VLDL. 2R means any form of alpha-tocopherol in which the 2 position is in the R conformation. The following table summarizes the forms of alpha-tocopherol that bind well to alpha-TTP, and those that don’t bind well to alpha-TTP.
Table 9.221 Alpha-tocopherol isomers and binding to alpha-TTP
Do not bind well to alpha-TTP | Bind well to alpha-TTP |
SRR | RRR |
SSR | RRS |
SSS | RSS |
SRS | RSR |
Other forms of vitamin E (gamma-tocopherol, tocotrienols) also don’t bind well to alpha-TTP and thus, are found in lower levels than alpha-tocopherol in the body. The following graph shows plasma vitamin E levels from a study in which subjects were given 150 mg each of RRR-alpha-tocopherol, all-rac-alpha-tocopherol, or gamma-tocopherol1.
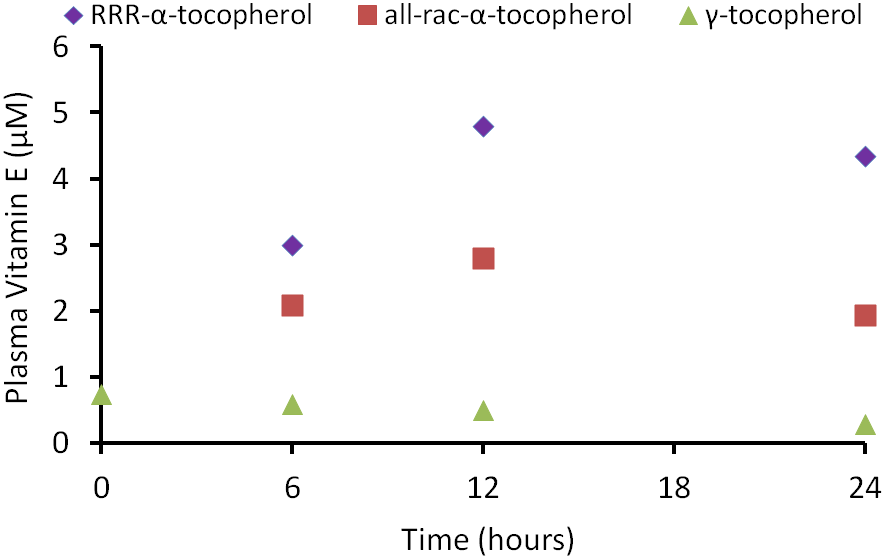
As you can see in the figure, there was a greater rise in the plasma alpha-tocopherol levels after receiving RRR-alpha-tocopherol vs. all-rac-alpha-tocopherol. This is not a surprise because approximately 50% of all-rac-alpha-tocopherol is 2R alpha-tocopherol that binds well with alpha-TTP. You can also see that the plasma gamma-tocopherol concentration is much lower than either natural or synthetic alpha-tocopherol.
From VLDL and subsequent lipoproteins, vitamin E reaches tissues, with most vitamin E in the body being found in the adipose tissue. There are 2 main routes of vitamin E excretion. The major route of excretion is through bile that is then excreted in feces. The second route is in the urine after vitamin E is chain-shortened in a process similar to beta-oxidation to make them more water-soluble.
Reference
- Traber MG, Elsner A, Brigelius-Floh R. (1998) Synthetic as compared with natural vitamin E is preferentially excreted as alpha-CEHC in human urine: Studies using deuterated alpha-tocopheryl acetates. FEBS Lett 437(1-2): 145-148.
9.23 Dietary Vitamin E & Amounts Found in Body
The best food sources of vitamin E are primarily oils and nuts. As you can see below, the forms of vitamin E that nuts and oils contain varies, with the two major forms being alpha and gamma-tocopherol. Soybean, corn, and flaxseed oils are good sources of gamma-tocopherol. Palm and canola oils contain almost equal amounts of alpha-tocopherol and gamma-tocopherol. Safflower oil, almonds, sunflower oil, and wheat germ oil are good sources of alpha-tocopherol. Beta-tocopherol and delta-tocopherol are found in lower levels in foods. Tocotrienols, for the most part, are not found in high levels in the diet. The amount of tocopherols in different nuts and oils are shown in the figure below.
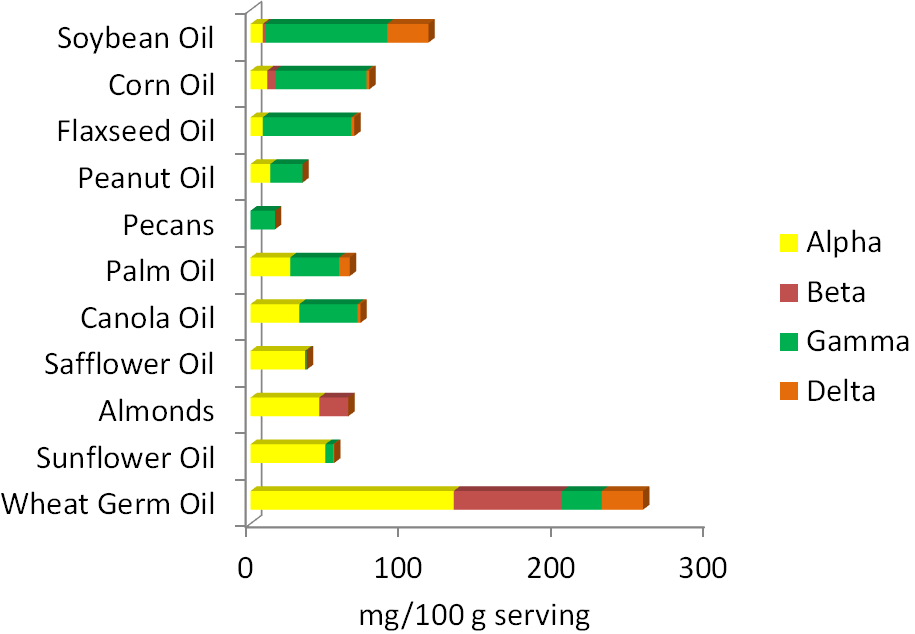
Three-fourths of the oil Americans consume is soybean oil. As a result, it is estimated that we consume 2-4 times more gamma-tocopherol than alpha-tocopherol. Europeans consume more olive, sunflower, and canola oil and thus are believed to consume at least 2 times more alpha-tocopherol than gamma-tocopherol1.
Despite Americans’ higher intake of gamma tocopherol compared to other countries, our serum concentrations do not differ much as illustrated in the table below.
Table 9.231 International serum gamma-tocopherol and alpha-tocopherol concentrations (uM/L)1
Location | Gamma-tocopherol | Alpha-tocopherol |
USA 1* | 2-7 | 15-20 |
USA 2 | 5.4 | 22.3 |
USA 3 | 2.5 | 21.8 |
Costa Rica | 2.7 | 28.6-31.8 |
France | 1.05-1.28 | 26.7 |
Ireland | 1.74-1.87 | 26.3 |
The Netherlands | 2.3 | 23.9-25.5 |
Spain | 0.88-1.14 | 27.4-28.3 |
Italy | 1.29 | 24.3 |
Sweden | 3.2 | 23.8 |
Lithuania | 1.64 | 21.7 |
Austria | 1.48 | 21.1 |
There are 3 different studies that have reported serum levels in the United States.
Tissue concentrations, for the most part, also indicate a greater accumulation of alpha-tocopherol than gamma-tocopherol as shown in the table below.
Table 9.232 Tissue gamma-tocopherol and alpha-tocopherol concentrations (nM/g)1
Tissue | Gamma-tocopherol | Alpha-tocopherol |
Skin | 180 | 127 |
Adipose | 176 | 440 |
Muscle | 107 | 155 |
Reference
- Wagner KH, Kamal-Eldin A, Elmadfa I. (2004) Gamma-tocopherol–an underestimated vitamin? Ann Nutr Metab 48(3): 169-188.
9.24 Vitamin E Deficiency & Toxicity
Vitamin E deficiency is extremely rare. Depletion studies require years on a vitamin E-deficient diet to cause deficiency1. Deficiency primarily occurs in people with lipid malabsorption problems or Ataxia with Isolated Vitamin E Deficiency (AVED). Individuals with AVED have a mutation in their alpha-TTP that prevents it from functioning correctly. The primary symptoms of vitamin E deficiency are neurological problems.
High levels of vitamin E intake do not result in a noted toxicity. However, higher levels of intake of alpha-tocopherol (like achieved by taking supplements) are associated with decreased blood coagulation. In particular, hemorrhagic stroke has been linked to high alpha-tocopherol intake levels. The link below shows that in this condition a blood vessel ruptures or leaks in the brain.
Web Link
Hemorrhagic Stroke (inactive link as of 05/18/2021) |
It is believed that this increased bleeding risk is due to a alpha-tocopherol metabolite that has anti-vitamin K activity. This potential antagonism will be described more in the vitamin K section.
References & Links
- DRI (2000) Dietary reference intakes for vitamin C, vitamin E, selenium, and carotenoids.
Link
Hemorrhagic Stroke – http://heart.arizona.edu/heart-health/preventing-stroke/lowering-risks-stroke
9.25 Vitamin E DRI & IUs
Before 2001, all forms of vitamin E contributed to the RDA, using a measure called alpha-tocopherol equivalents. In 2001, the Dietary Reference Intake (DRI) committee decided only 2R forms of alpha-tocopherol should be used to estimate the requirement, because these forms bind to alpha-TTP. Thus, other forms of vitamin E (gamma-tocopherol, tocotrienols etc.) do not count toward the requirement and the unit is now mg of alpha-tocopherol. As a result, soybean, corn, and flaxseed oils, which are good sources of gamma-tocopherol, are no longer considered to be good sources of vitamin E. The figure below is a reminder of the tocopherol content of different nuts and oils.
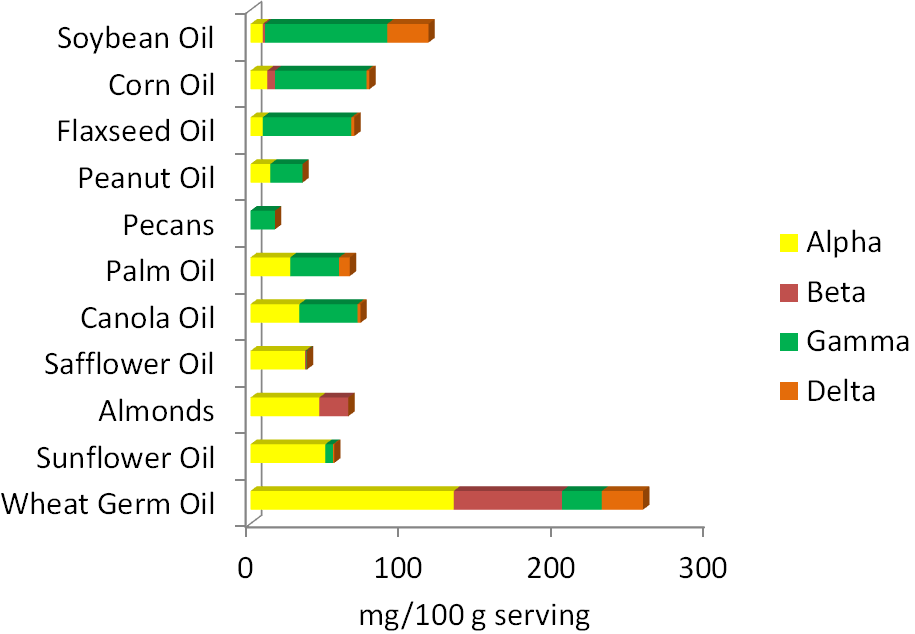
Another level of complexity is added by the introduction of international units (IU). IUs are a unit that are used to describe the bioactivity of different compounds, including 4 vitamins: A, D, E, and C. It would be less confusing if these units were not used. However, most supplements use IUs. IUs are not as common on food items.
For vitamin E, IUs are specific for alpha-tocopherol and adjusted for the molecular weight of the different forms (alpha-tocopherol acetate etc.). The conversion factors for converting IU to mg of alpha-tocopherol are:
0.67 for RRR-alpha-tocopherol (and its esters)
0.45 for all-rac-alpha-tocopherol (and its esters)
Here are some example calculations showing how to use these conversion factors:
Example 1. For a supplement containing 100 IU of RRR-alpha tocopherol:
100 IU X 0.67 = 67 mg alpha-tocopherol
Example 2. For a supplement containing 100 IU of all-rac-alpha tocopherol:
100 IU X 0.45 = 45 mg alpha-tocopherol2,3
References & Links
- Wagner KH, Kamal-Eldin A, Elmadfa I. (2004) Gamma-tocopherol–an underestimated vitamin? Ann Nutr Metab 48(3): 169-188.
- DRI (2000) Dietary reference intakes for vitamin C, vitamin E, selenium, and carotenoids.
- https://ods.od.nih.gov/factsheets/VitaminE-HealthProfessional/
9.3 Vitamin C
Vitamin C is well-known for being a water-soluble antioxidant. Humans are one of the few mammals that don’t synthesize vitamin C, making it an essential micronutrient. Other mammals that don’t synthesize vitamin C include primates, guinea pigs, and other less prevalent species1.
Vitamin C’s scientific names are ascorbic acid or ascorbate and the oxidized form is dehydroascorbic acid or dehydroascorbate. The structure of vitamin C is shown below.
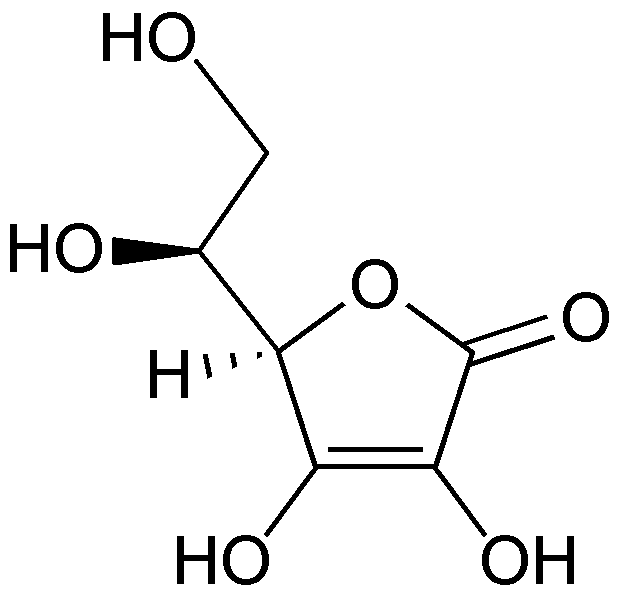
When ascorbic acid is oxidized, it forms semidehydroascorbate (1 degree of oxidation) and then dehydroascorbate (2 degrees of oxidation). The structure of dehydroascorbic acid is shown below.
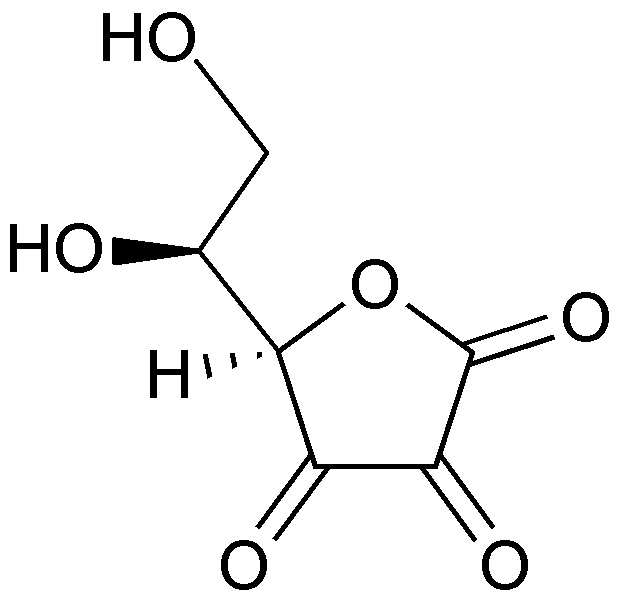
The figure below shows the reaction through which ascorbic acid can stabilize or quench 2 free radicals. The 2 circled hydrogens are lost and replaced by double bonds when ascorbic acid is oxidized to dehydroascorbic acid. Reducing dehydroascorbic acid back to ascorbic acid is the opposite reaction.
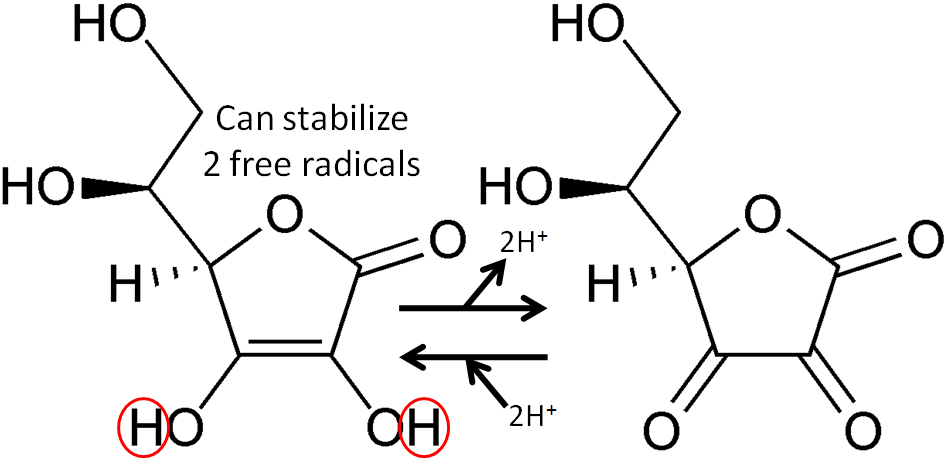
Ascorbic acid is believed to be a part of an antioxidant network (shown below) where it is oxidized to reduce alpha-tocopherol radicals. Dehydroascorbic acid can be reduced by thioredoxin reductase, a selenoenzyme, to regenerate ascorbic acid.
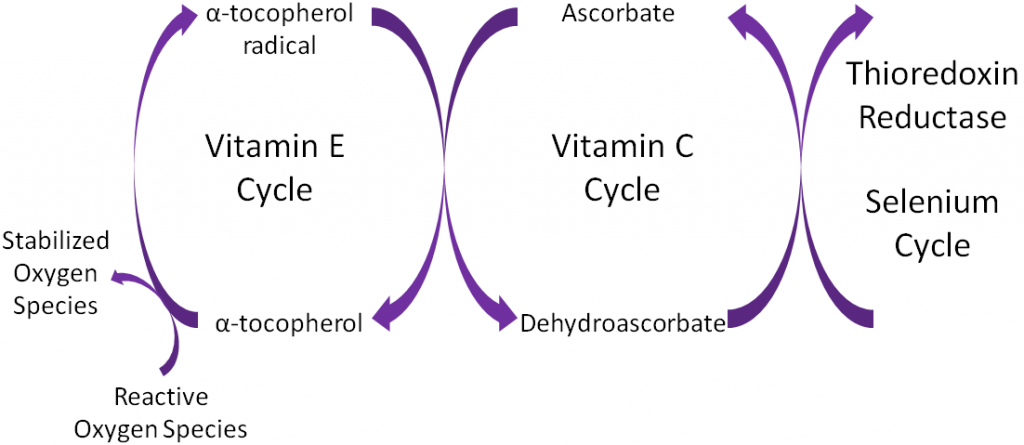
Subsections:
9.31 Absorption and Tissue Accumulation of Vitamin C
9.32 Enzymatic Functions
9.33 Vitamin C Deficiency – Scurvy
9.34 Vitamin C Toxicity, Linus Pauling, & the Common Cold
References & Links
- Stipanuk MH. (2006) Biochemical, physiological, & molecular aspects of human nutrition. St. Louis, MO: Saunders Elsevier.
- http://en.wikipedia.org/wiki/File:Ascorbic_acid_structure.png
- http://en.wikipedia.org/wiki/File:Dehydroascorbic_acid.png
- Packer L, Weber SU, Rimbach G. (2001) Molecular aspects of alpha-tocotrienol antioxidant action and cell signalling. J Nutr 131(2): 369S-373S.
9.31 Vitamin C Absorption & Tissue Accumulation
Vitamin C is found in foods primarily as ascorbic acid (80-90%), but dehydroascorbic acid (10-20%) is also present. The bioavailability of vitamin C is high at lower doses as shown below, but drops to less than 50% at higher doses.
Table 9.311 Bioavailability of vitamin C1
Dose (mg) | % Bioavailability |
200 | 112 |
500 | 73 |
1250 | 49 |
Ascorbic acid is actively absorbed by the sodium vitamin C cotransporter (SVCT) 1. This active transport is driven by the sodium electrochemical gradient created by sodium-potassium ATPase. Ascorbic acid then diffuses into the capillary and ultimately enters general circulation. Vitamin C generally circulates as ascorbic acid.
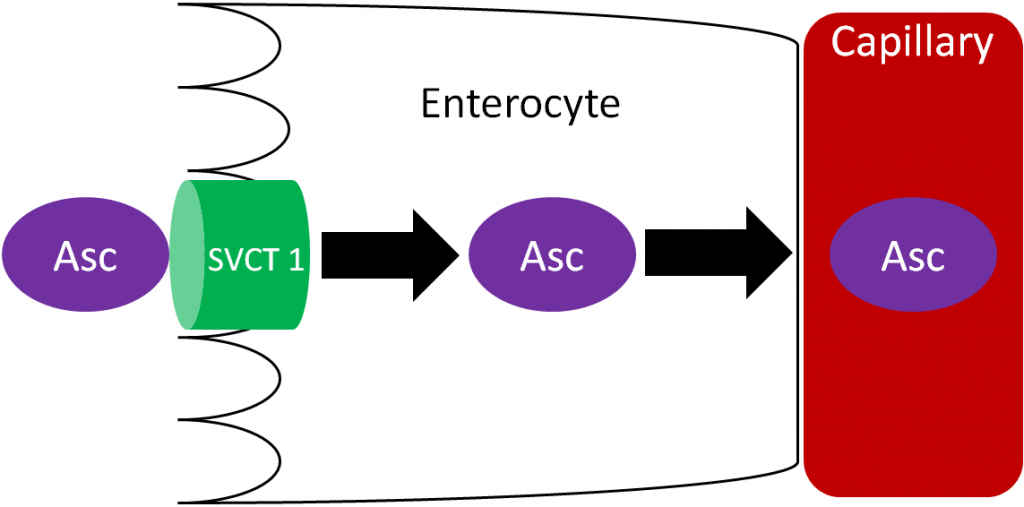
Accumulation
Most water-soluble vitamins are not stored in the body. Vitamin C is not stored, but is accumulated in certain tissues in the body where it can be 5-100 times higher than found in the plasma2. The table below shows the concentrations of vitamin C in different tissues and fluids.
Table 9.312 Human tissue & fluid ascorbic acid concentrations1
Organ/Tissue | Vitamin C Concentration* | Organ/Tissue | Vitamin C Concentration* |
Pituitary Gland | 40-50 | Lungs | 7 |
Adrenal Gland | 30-40 | Skeletal Muscle | 3-4 |
Eye Lens | 25-31 | Testes | 3 |
Liver | 10-16 | Thyroid | 2 |
Brain | 13-15 | Cerebrospinal Fluid | 3.8 |
Pancreas | 10-15 | Plasma | 0.4-1 |
Spleen | 10-15 | Saliva | 0.1-9.1 |
Kidneys | 5-15 |
* mg/100 g wet tissue, mg/100 mL fluids
How does the body accumulate such high levels of vitamin C? There are 2 primary mechanisms:
- Ascorbic Acid (Ascorbate) uptake using sodium-dependent vitamin C transporter (SVCT) 1 or 2
- Ascorbic Acid (Ascorbate) Recycling
Ascorbic Acid (Ascorbate) transport using sodium-dependent vitamin C transporter (SVCT) 1 or 2
As shown below, SVCT 1 and SVCT 2 transport ascorbic acid or ascorbate into the cell against the concentration gradient (represented by the orange wedge in the figure below). Like absorption, this uptake is driven by the action of sodium-potassium ATPase. This mechanism is saturable, meaning that at high concentrations it reaches a threshold where it cannot take up ascorbic acid any faster. Thus, there is a limit to how much can be taken up through this mechanism3.
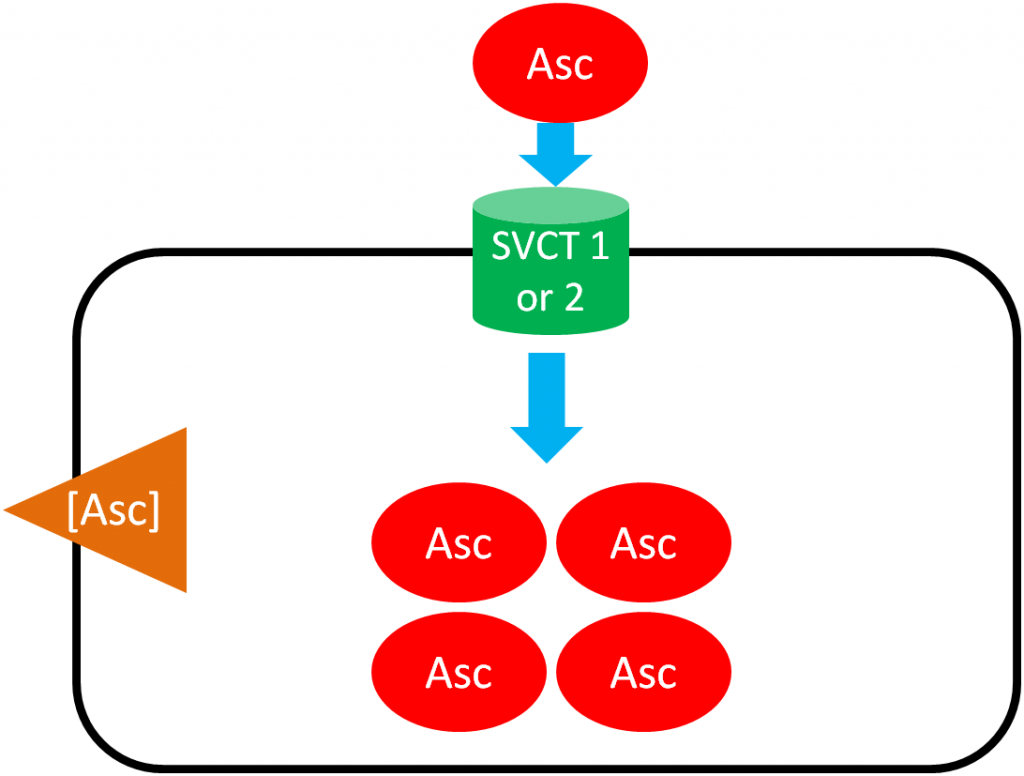
Ascorbic Acid (Ascorbate) Recycling
In ascorbic acid recycling, ascorbic acid is oxidized to dehydroascorbic acid (DHA). DHA is then transported into the cell moving with its concentration gradient using GLUT1 or 3. Once inside the cell, DHA is reduced back to ascorbic acid, thus maintaining the DHA gradient. As a result, the cell is able to accumulate high levels of ascorbic acid3. The figure below depicts ascorbic acid recycling.
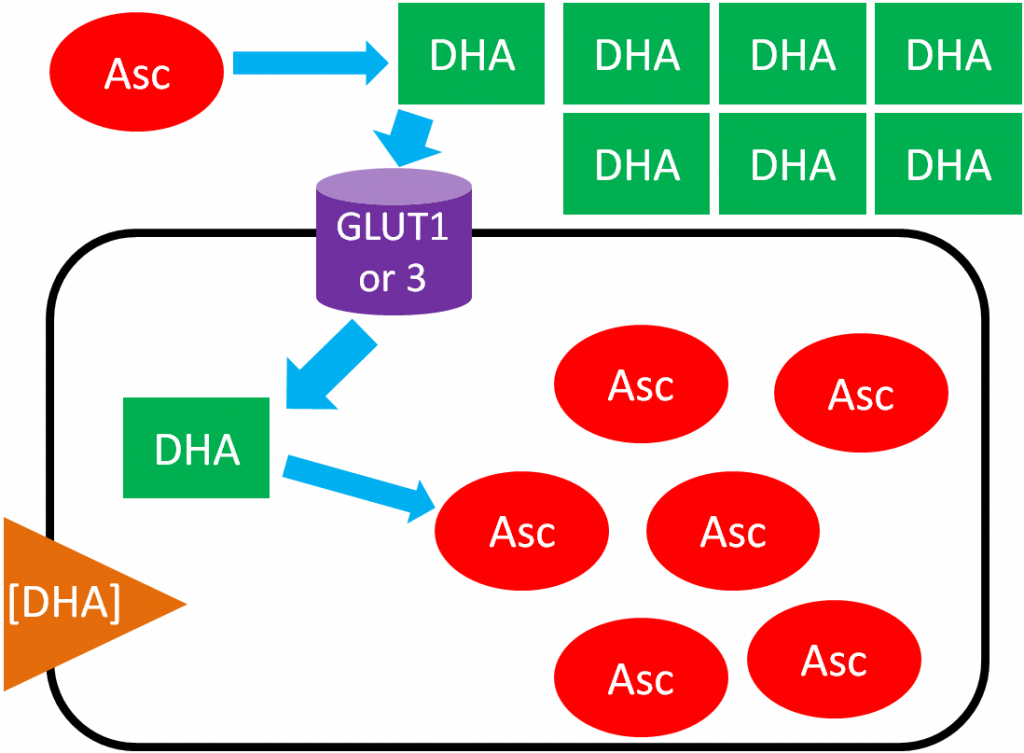
References & Links
- Shils ME, Shike M, Ross AC, Caballero B, Cousins RJ, editors. (2006) Modern nutrition in health and disease. Baltimore, MD: Lippincott Williams & Wilkins.
- Stipanuk MH. (2006) Biochemical, physiological, & molecular aspects of human nutrition. St. Louis, MO: Saunders Elsevier.
- Li Y, Schellhorn H. (2007) New developments and novel therapeutic perspectives for vitamin C. J Nutr 137(10): 2171-2184.
9.32 Enzymatic Functions
In addition to its antioxidant function, vitamin C is also a cofactor for a number of enzymes. The enzymes proline hydroxylase and lysyl hydroxylase are important in the formation of the protein collagen. Hydroxylase means that the enzymes add alcohol (hydroxyl, -OH) to the amino acids proline and lysine, as shown below.
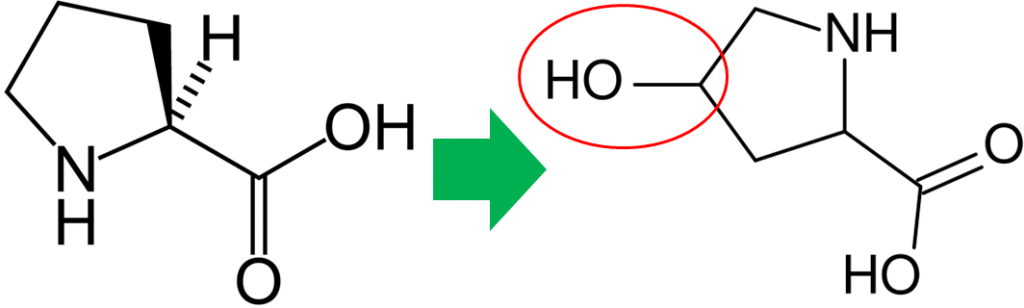
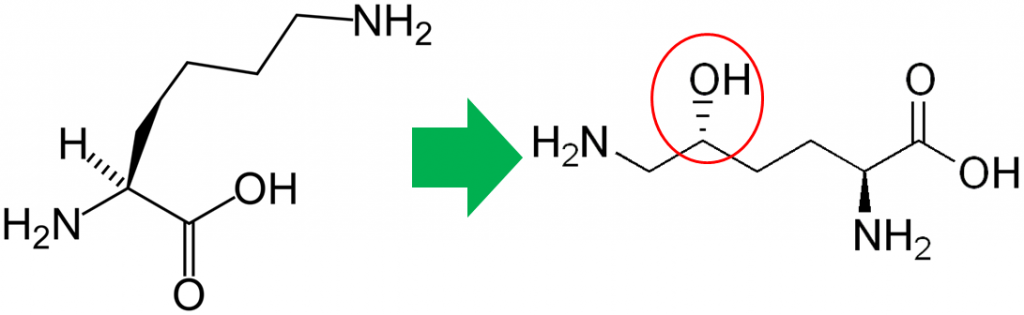
As shown below, prolyl and lysyl hydroxylases require ferrous iron (Fe2+) to function. But in the course of the hydroxylating proline or lysine, ferrous iron (Fe2+) is oxidized to ferric iron (Fe3+). Ascorbic acid is required to reduce Fe3+ to Fe2+, forming semidehydroascorbic acid in the process. With Fe2+, the enzyme is then able to continue to hydroxylate proline and lysine.
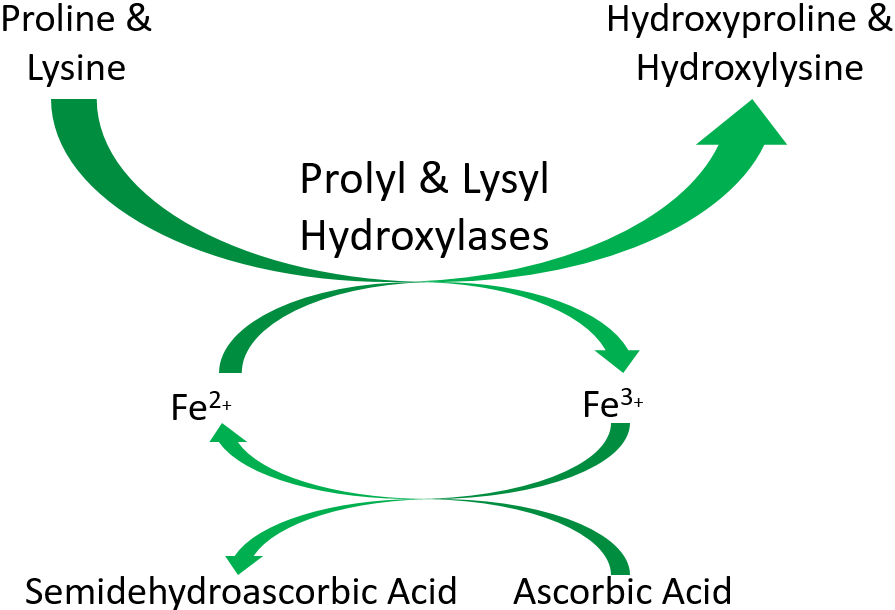
Why should you care about collagen formation? Because collagen is estimated to account for 30% or more of total body proteins5. Collagen contains a number of hydroxylated prolines and lysines that are needed for collagen strands to properly cross-link. This cross-linking is important for collagen to wind together like a rope, forming the strong triple helix known as tropocollagen. This process is shown in the following animation, as well as in the figure below.
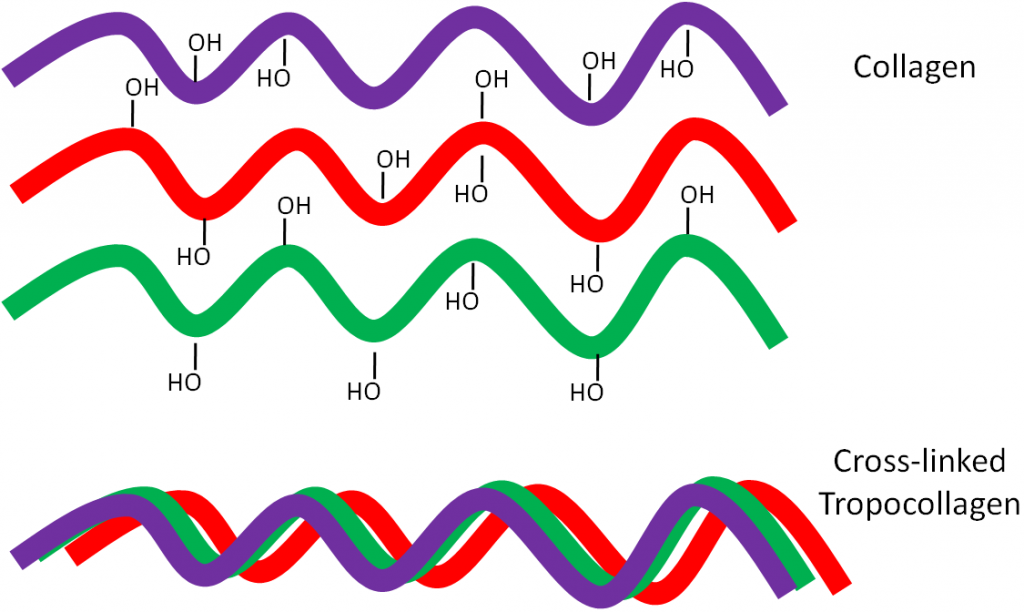
But if there isn’t enough ascorbic acid available, the collagen strands are underhydroxylated and instead of forming strong tropocollagen, the underhydroxylated collagen is degraded as shown below.
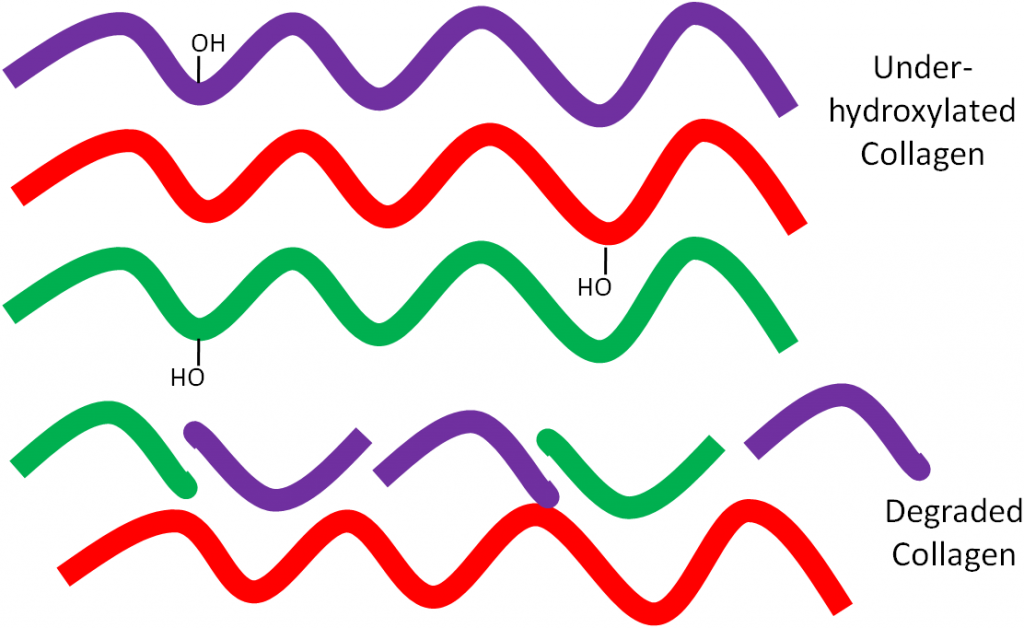
This weak collagen then results in the symptoms seen in the vitamin C deficiency, scurvy, that will be discussed in the next subsection.
Ascorbic Acid is also needed for:
Carnitine synthesis
Tyrosine synthesis and catabolism
Serotonin (neurotransmitter) synthesis
Other hormone and neurotransmitter synthesis6
The figure below shows how ascorbic acid is needed for dopamine hydroxylase, which ultimately produces the hormone epinephrine.
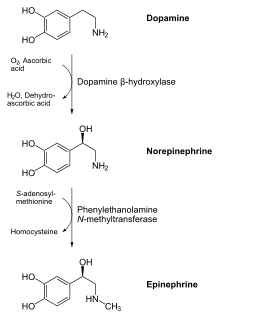
References & Links
- https://en.wikipedia.org/wiki/Proline#/media/File:Prolin_-_Proline.svg
- http://en.wikipedia.org/wiki/File:Hydroxyproline_structure.svg
- https://en.wikipedia.org/wiki/Lysine#/media/File:L-lysine-monocation-2D-skeletal.png
- http://en.wikipedia.org/wiki/File:Hydroxylysine.png
- Di Lullo G, Sweeney S, Korkko J, Ala-Kokko L, San Antonio J. (2002) Mapping the ligand-binding sites and disease-associated mutations on the most abundant protein in the human, type I collagen. The Journal of Biological Chemistry 277(6): 4223-4231.
- Gropper SS, Smith JL, Groff JL. (2008) Advanced nutrition and human metabolism. Belmont, CA: Wadsworth Publishing.
- http://en.wikipedia.org/wiki/File:Catecholamines_biosynthesis.svg
9.33 Vitamin C Deficiency (Scurvy)
Why should you care about the functions of vitamin C? Because they explain the symptoms of vitamin C deficiency. While it is rare in the United States, vitamin C deficiency, known as scurvy, displays symptoms that are a result of weak collagen, that in turn, weakens connective tissue throughout the body. Symptoms of scurvy include bleeding gums, pinpoint hemorrhages, and corkscrew hairs as shown in the figure and link below.
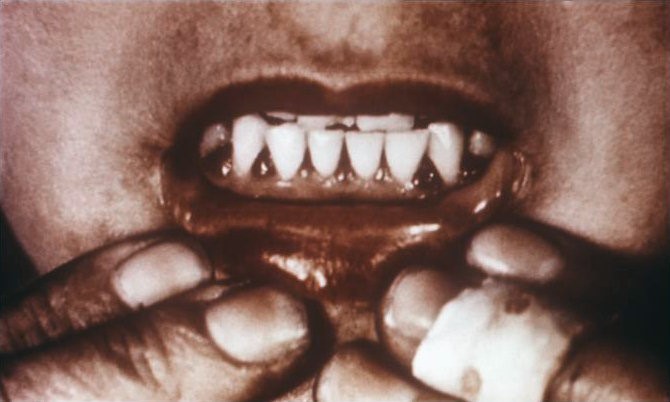
Web Link |
Additional symptoms include impaired wound and fracture healing, easy bruising, and loose or decaying teeth. Scurvy can be fatal if not treated. Scurvy was the first discovered nutrition deficiency in 1746 by James Lind, who is shown below.
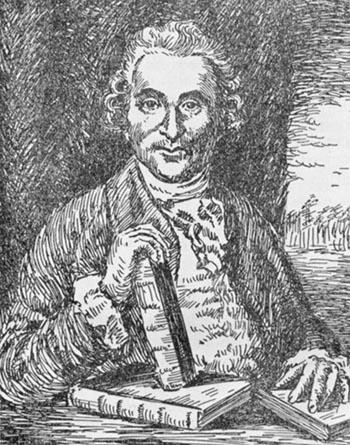
Keep in mind as you read the description below that suggesting scurvy was due to a diet deficiency, at that time, sounded like flu was caused by not consuming green beans. Sounds pretty crazy, right?
Lind was a surgeon on a British navy ship. Frequently during voyages the sailors would develop scurvy for reasons that weren’t understood at the time. It was known that citrus fruits could cure or prevent scurvy, but it was believed this was due to their acidity. Lind performed clinical trials comparing citrus juice to dilute sulfuric acid and vinegar and found that only citrus juice caused the sailors to recover, as depicted in the link below. As a result of the discovery, the British sailors became known as “Limeys” because they would drink lime juice to prevent the development of the disease4.
Web Link |
References & Links
- http://en.wikipedia.org/wiki/File:Scorbutic_gums.jpg
- Gropper SS, Smith JL, Groff JL. (2008) Advanced nutrition and human metabolism. Belmont, CA: Wadsworth Publishing.
- http://en.wikipedia.org/wiki/File:James_lind.jpg
- Carpenter K. (2003) A short history of nutritional science: Part 3 (1912-1944). J Nutr 133(10): 638-645.
Links
Corkscrew Hairs – http://www.nlm.nih.gov/medlineplus/ency/imagepages/2345.htm
Curing Scurvy – http://ericbakker.com/wp-content/uploads/2015/05/scurvy.png
9.34 Vitamin C Toxicity, Linus Pauling & the Common Cold
Vitamin C does not have a toxicity per se, but in some people over 2 grams/day can lead to diarrhea and gastrointestinal distress. In addition, high intake of vitamin C increases excretion of uric acid (urate) and oxalic acid (oxalate). The structure of these 2 compounds are shown below.
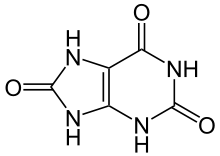
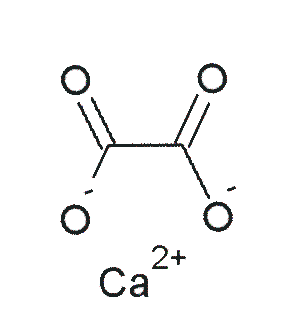
These compounds are the primary components of 2 types of kidney stones3. The figures below show the most common sites of pain in someone with kidney stones.
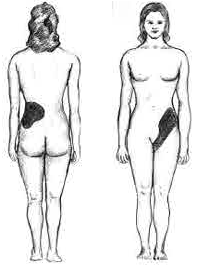
The following video describes what kidney stones are and the symptoms that can occur if someone has kidney stones. The second link shows some pictures of kidney stones.
Web Links |
Calcium oxalate is one of the primary forms of kidney stones with uric acid stones being more rare3. However, a link between excretion of these compounds and actual stone formation hasn’t been established. Nevertheless, high-dose vitamin C supplementation should be approached with some caution, since it is not clear whether it increases the risk of forming kidney stones5.
Linus Pauling and the common cold
The person who popularized taking megadoses of vitamin C was Dr. Linus Pauling. Dr. Pauling was a chemist, and is the only person to receive 2 unshared Nobel Prizes. The Nobel Prize is a prestigious award, and Dr. Pauling was close to solving the structure of DNA. This would have likely netted him another Nobel prize, but Watson and Crick beat him to it.
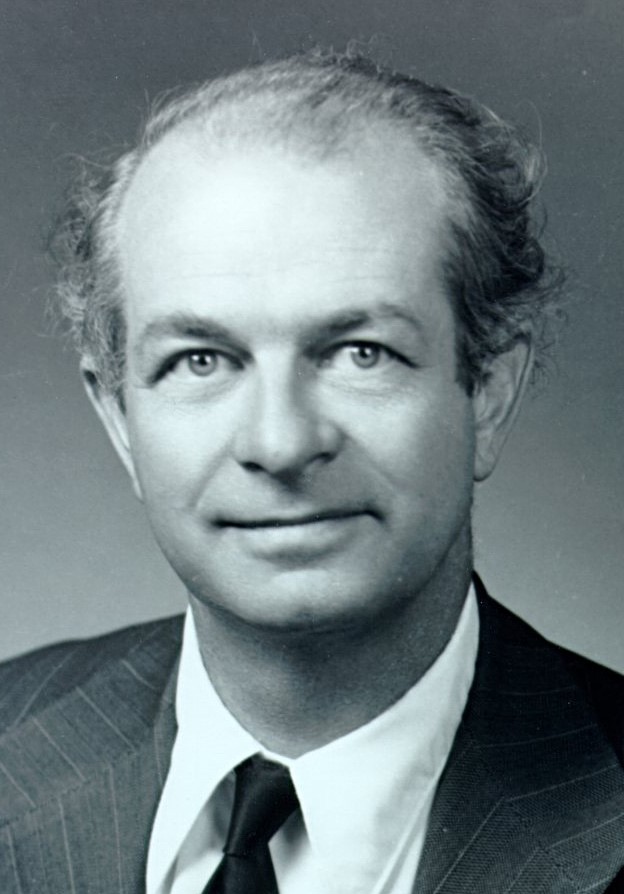
Later in his life Pauling became convinced that megadoses of vitamin C could prevent the common cold. In 1970 his book Vitamin C and the Common Cold was released and became a bestseller. Later he came to believe that vitamin C could prevent cardiovascular disease, cancer, and combat aging7. However, critics of his beliefs countered that all megadose supplementation was doing was creating “expensive urine”. This refers to the fact that the RDA is only 75-90 mg/day for adults and Pauling recommended taking 1-2 grams of vitamin C daily8. Thus, with vitamin C being water-soluble, most of the vitamin C that people on the regimen were paying to consume was being excreted in the urine, thus making it “expensive”.
A recent review of vitamin C and colds found that that routine megadoses of vitamin C do not reduce the risk of the common cold in most individuals. However, there is some evidence that it might benefit people exposed to brief periods of severe physical exercise (marathon runners) or cold environments (skiers and soldiers in subarctic conditions). There has been little research conducted in children, so it is not known whether vitamin C supplementation is beneficial in this age group9.
References & Links
- http://en.wikipedia.org/wiki/File:Harns%C3%A4ure_Ketoform.svg
- http://en.wikipedia.org/wiki/File:Calcium_oxalate.png
- https://www.niddk.nih.gov/health-information/urologic-diseases/kidney-stones
- https://commons.wikimedia.org/wiki/File:Pos-renal.png
- Massey L, Liebman M, Kynast-Gales S. (2005) Ascorbate increases human oxaluria and kidney stone risk. J Nutr 135(7): 1673-1677.
- https://www.flickr.com/photos/oregonstateuniversity/5711642694
- http://lpi.oregonstate.edu/lpbio/lpbio2.html
- http://www.health.harvard.edu/newsletter_article/excerpts_from_vitamin_c_and_the_common_cold_by_linus_pauling
- Hemilä H, Chalker E, Douglas B. Vitamin C for preventing and treating the common cold. Cochrane Database of Systematic Reviews 2007, Issue 3. Art. No.: CD000980. DOI: 10.1002/14651858.CD000980.pub3.
Video
Kidney Stones – https://www.youtube.com/watch?v=16ewFJ-iQtw
Links
Kidney Stones – http://www.herringlab.com/photos/index.html
9.4 Selenium
Selenium can be divided into 2 categories: organic and inorganic. The organic forms contain carbon, while the inorganic forms do not. The primary inorganic forms of selenium are selenite (SeO3) and selenate (SeO4). Selenite and selenate are not commonly found alone in nature; they are usually complexed with sodium to form sodium selenite (Na2SeO3) and sodium selenate (Na2SeO4)1.
Selenomethionine is the most common organic form of selenium. The structure of selenomethionine is shown above the structure of the amino acid methionine in the figure below.
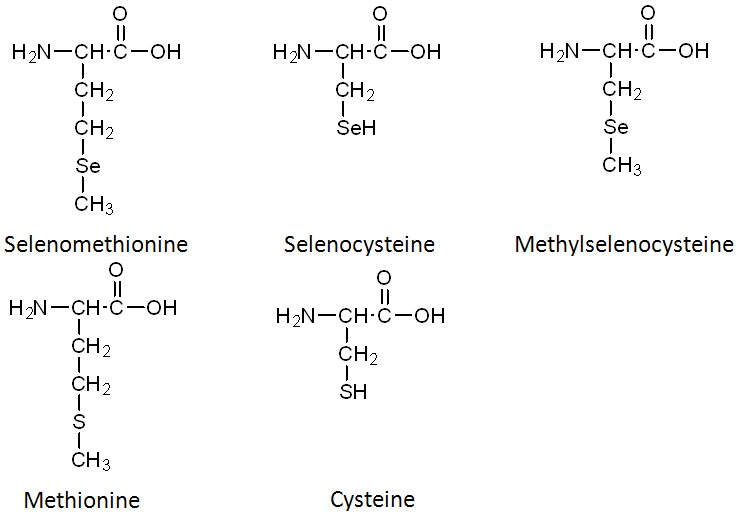
In comparing the structures of selenomethionine or methionine, you can see that the only difference is that selenium has been substituted for the sulfur (S) atom in methionine. Selenocysteine is considered the 21st amino acid by some, because there is a codon that directs its insertion into selenoproteins. Like selenomethionine versus methionine, the only difference between selenocysteine and cysteine is the substitution of selenium for sulfur. The last organic form is methylselenocysteine (aka Se-methylselenocysteine). Notice that its structure is like selenocysteine, but with a methyl group added (like the name suggests).
The selenium content of plants is dependent on the soil where they are grown. As shown below, soil selenium levels vary greatly throughout the United States, meaning that the selenium content of plant foods also greatly vary.
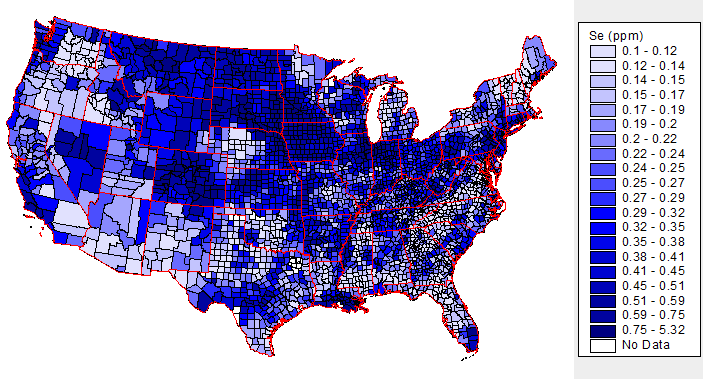
The above map is interactive, so to see the soil selenium levels in a certain county or state, click on it in the link below.
Web Link |
Inorganic forms of selenium are commonly used in supplements. Selenomethionine is the most common organic form of selenium in supplements and food. It is found in cereal grains such as wheat, corn, and rice as well as soy. Yeast are typically used to produce selenomethionine for supplements.
It should be noted that selenomethionine accumulates at much higher levels in the body than other forms of selenium. This is because it can be nonspecifically incorporated into body proteins in place of methionine. However, despite accumulating at higher levels, selenomethionine is less effective than the methylselenocysteine in decreasing cancer incidence or growth in animal models3. However, methylselenocysteine is not commonly consumed, because it is a form that plants accumulate to prevent selenium from becoming toxic to themselves. Thus, plants need to be grown in the presence of high selenium levels to accumulate meaningful amounts of methylselenocysteine.
Subsections:
9.41 Selenoprotein
9.42 Selenium Absorption, Excretion, Toxicity & Its Questionable Deficiency
References & Links
- Stipanuk MH. (2006) Biochemical, physiological, & molecular aspects of human nutrition. St. Louis, MO: Saunders Elsevier.
- http://tin.er.usgs.gov/geochem/doc/averages/se/usa.html
- Lindshield B, Ford N, Canene-Adams K, Diamond A, Wallig M, et al. (2010) Selenium, but not lycopene or vitamin E, decreases growth of transplantable dunning R3327-H rat prostate tumors. PloS One 5(4): e10423-e10423.
Link
USGS Soil Selenium Levels – http://tin.er.usgs.gov/geochem/doc/averages/se/usa.html
9.41 Selenoproteins
As mentioned earlier, selenium’s antioxidant function is not due to the mineral itself, but a result of selenoproteins. This is illustrated in the figure below, where the different colored circles represent amino acids in the crescent shaped enzyme. In most enzymes, the mineral is a cofactor that is external to the enzyme, as shown on the left. Selenoenzymes contain selenocysteine as an amino acid in the active site of the enzyme. Thus, in selenoenzymes, selenium does not serve as a cofactor, which is different than most minerals required for enzyme function.
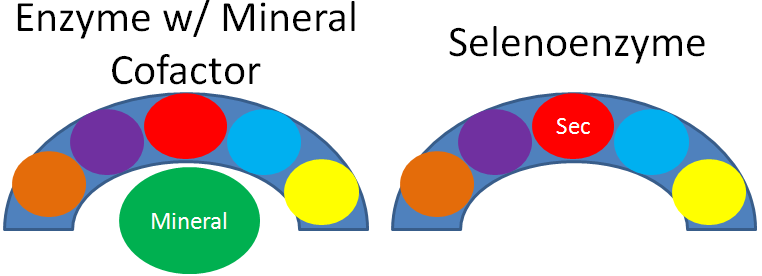
25 human selenoproteins, containing the amino acid selenocysteine, have been identified. The following table lists these selenoproteins along with their function.
Table 9.411 The 25 Human Selenoproteins1-3
Selenoprotein | Function |
Glutathione peroxidase 1 (GPX1) | Antioxidant enzyme |
Glutathione peroxidase 2 (GPX2) | Antioxidant enzyme |
Glutathione peroxidase 3 (GPX3) | Antioxidant enzyme |
Glutathione peroxidase 4 (GPX4) | Antioxidant enzyme |
Glutathione peroxidase 6 (GPX6) | Antioxidant enzyme |
Iodothyronine 5′-deiodinase-1 (DI1) | Plasma T3 production |
Iodothyronine 5′-deiodinase-2 (DI2) | Local T3 production |
Iodothyronine 5′-deiodinase-3 (DI3) | T3 degradation |
Thioredoxin reductase (TR1) | Antioxidant enzyme |
Thioredoxin reductase (TR2) | Antioxidant enzyme |
Thioredoxin reductase (TR3) | Antioxidant enzyme |
Selenophosphate synthetase 2 (SPS2) | Selenophosphate synthesis |
Selenoprotein 15 (Sep15) | Unknown |
Selenoprotein H (SepH) | Unknown |
Selenoprotein I (SepI) | Unknown |
Selenoprotein K (SepK) | Unknown |
Selenoprotein M (SepM) | Unknown |
Selenoprotein N (SepN) | Unknown |
Selenoprotein O (SepO) | Unknown |
Selenoprotein P (SepP) | Unknown |
Selenoprotein R (SepR) | Unknown |
Selenoprotein S (SepS) | Unknown |
Selenoprotein T (SepT) | Unknown |
Selenoprotein V (SepV) | Unknown |
Selenoprotein W (SepW) | Unknown |
Hopefully from looking at the table, you see that the glutathione peroxidase enzymes and thioredoxin reductases are antioxidant enzymes. The iodothyronine 5′-deiodinases are involved in the metabolism of thyroid hormones, which will be discussed further in the iodine section. For the vast majority of the other selenoproteins, their function isn’t known, so they were named selenoprotein and given a letter. As described earlier and shown below, glutathione peroxidase converts hydrogen peroxide into water.
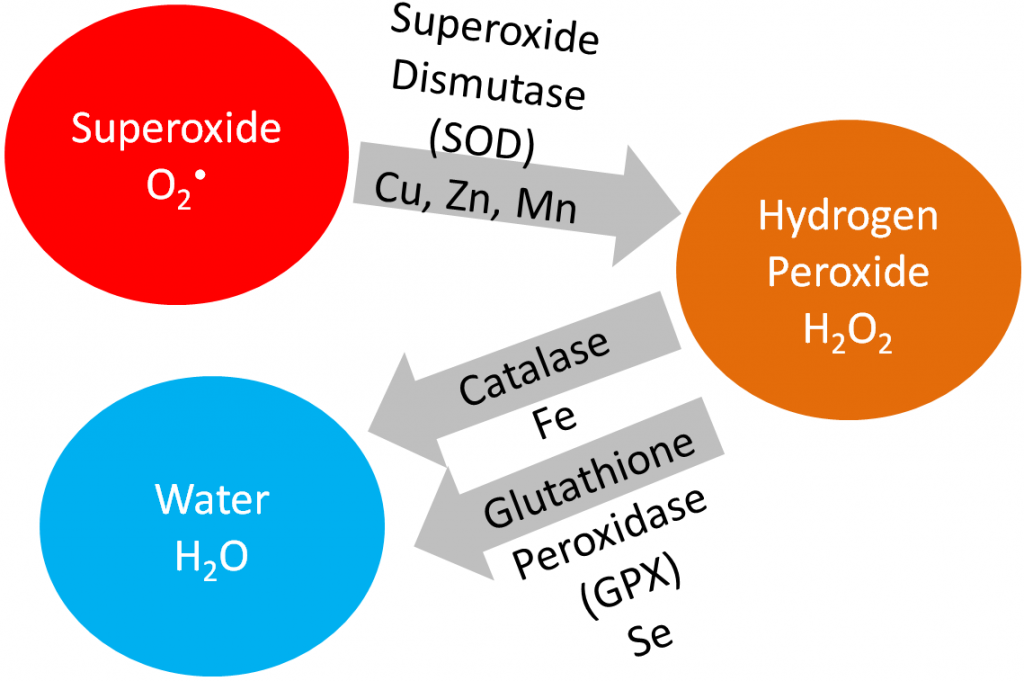
Remember that thioredoxin reductase can regenerate ascorbate from dehydroascorbate in the theorized antioxidant network (shown below).
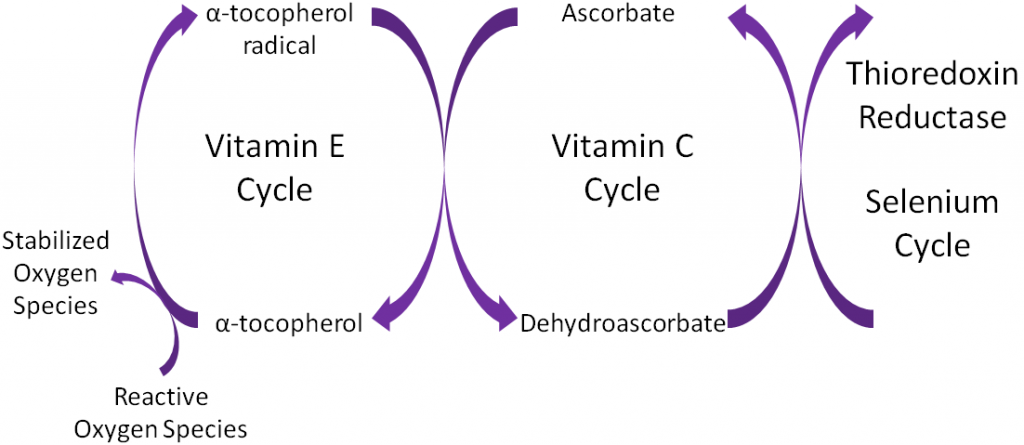
References & Links
- Gladyshev V, Kryukov G, Fomenko D, Hatfield D. (2004) Identification of trace element-containing proteins in genomic databases. Annu Rev Nutr 24: 579-596.
- Beckett G, Arthur J. (2005) Selenium and endocrine systems. J Endocrinol 184(3): 455-465.
- Stipanuk MH. (2006) Biochemical, physiological, & molecular aspects of human nutrition. St. Louis, MO: Saunders Elsevier.
- Packer L, Weber SU, Rimbach G. (2001) Molecular aspects of alpha-tocotrienol antioxidant action and cell signalling. J Nutr 131(2): 369S-373S.
9.42 Selenium Absorption, Excretion, Toxicity & Its Questionable Deficiency
Selenium is highly absorbed. Thus, selenium levels in the body are not regulated by absorption, but rather by urinary excretion. Organic selenium forms may be absorbed slightly better than inorganic forms, as one study found that 98% of a dose of selenomethionine was absorbed, compared to 84% of selenite1.
Selenium is primarily excreted in the urine, but at high levels it can be expired, producing garlic odor breath.
Selenium toxicity can be a problem, especially for animals living in or around a body of water in an area with high soil selenium levels. This is because runoff from the soil causes selenium to collect in the water in high levels and then starts working its way up the food chain and causing problems, as shown in the following link.
Web Link |
In humans, the initial symptoms are nausea, fatigue, and diarrhea. If continued, the person may
develop hair and nail brittleness, rash or skin lesions, and nervous system abnormalities.
The questionable selenium deficiency is Keshan disease. This disease occurred primarily in the mountainous regions of China, causing heart lesions. Below is a topographical map of China to give you an idea of what areas are mountainous.
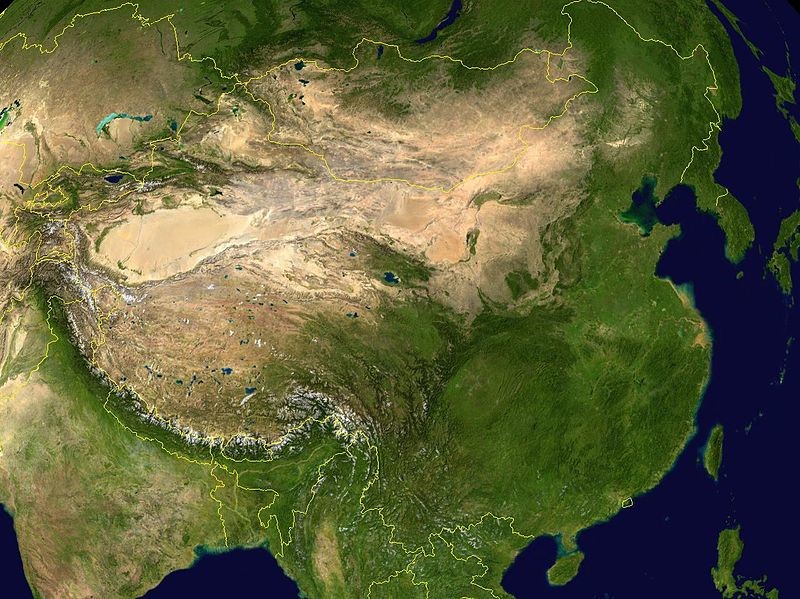
You can see in the link below the areas where Keshan disease occurred. For the most part, you can see that these 2 areas overlay.
Web Links |
However, sodium selenate supplementation failed to totally eradicate Keshan disease like you would expect if it was caused by selenium deficiency. The incidence of Keshan disease also fluctuated seasonally and annually, which is unusual for a deficiency and more consistent of an infectious disease. Research found coxsackievirus in the heart of Keshan disease victims. They isolated this virus and used it to perform the experiment illustrated below.
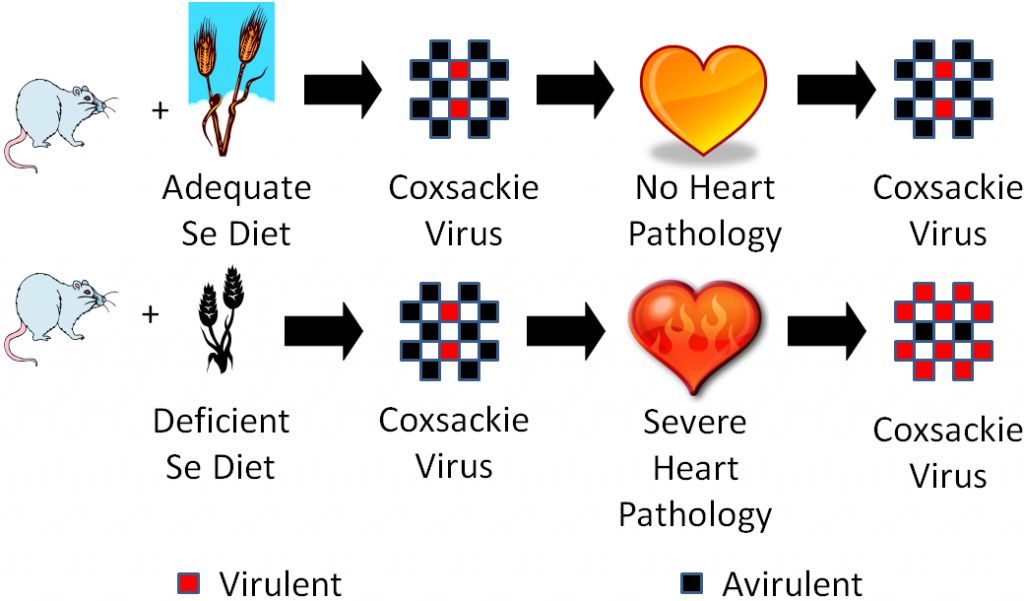
One group of mice was fed an adequate selenium diet and another group a deficient selenium diet. They were then infected with coxsackievirus that was mostly avirulent, but also contained some virulent viruses. A virulent virus is one that causes a disease, and an avirulent virus is one that doesn’t cause a disease (some vaccines use avirulent viruses). After a period of time, they found that the selenium deficient animals developed severe heart pathology, while the selenium adequate animals did not develop heart pathology. They then isolated the virus from the hearts of the mice from both groups and found that the coxsackievirus from the deficient animals hearts had become mostly virulent3. They then took it one step further as shown in the figure below.
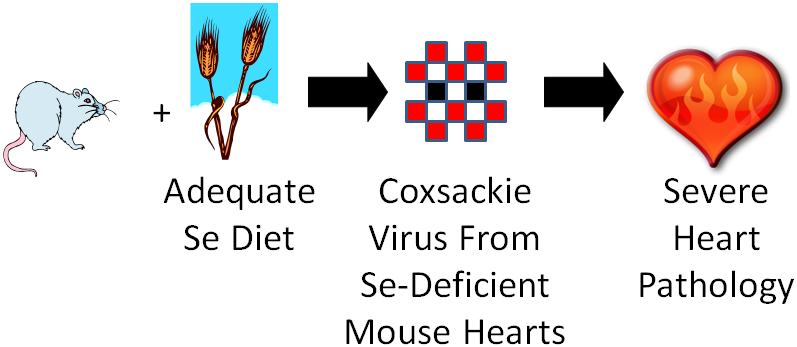
They took the isolated virus from the selenium-deficient mouse hearts and infected selenium adequate animals with it. The selenium adequate animals developed the severe heart pathology like the selenium-deficient animals had previously.
What’s going on? They found mutations in the virus from the selenium-deficient animals that they believe caused it to become virulent. They believe that high oxidative stress in these animals leads to mutations in the virus, causing it to become virulent.
Who cares? Research has found similar results with vitamin E. Researchers are also examining the effects on other viruses such as influenza (flu) and HIV. If they find a similar phenomenon occurring in other viruses, it means that you and your friend who eats a horrible diet (eats no fruit and vegetables) could be exposed to a virus. You don’t know you were exposed because your immune system fights off the virus. However, your friend gets sick. He/she can serve as a host in which the virus mutates making it more virulent, which when you’re exposed a second time, may make you sick.
References & Links
- Stipanuk MH. (2006) Biochemical, physiological, & molecular aspects of human nutrition. St. Louis, MO: Saunders Elsevier.
- http://en.wikipedia.org/wiki/File:China_100.78713E_35.63718N.jpg
- Beck M, Handy J, Levander O. (2004) Host nutritional status: The neglected virulence factor. Trends Microbiol 12(9): 417-423.
Links
Selenium Toxicity – http://www.sci.sdsu.edu/salton/SeTooMuchTooLittle.html
Distribution of Keshan Disease – http://pubs.acs.org/cen/80th/selenium.html