V. Carbohydrates, Metabolism
New Terms
Adenosine Triphosphate (ATP)
Acetyl Coenzyme A (CoA)
Electron transport chain
Flavin Adenine Dinucleotide (FAD)
Glycolysis
Nicotinamide Adenine Dinucleotide (NAD)
Pyruvate
Pyruvate dehydrogenase
Tricarboxylic Acid (TCA) cycle
Chapter Objectives
- Introduce the fate of absorbed glucose and volatile fatty acids
- To provide information on bioenergetics of glucose catabolism in the animal body
Why Do Animals Need Energy?
Energy is defined as the “ability to do work”. Animals need energy to carry out all the body processes (e.g., nutrient transport, synthesis, muscle contraction) required to maintain life. Without energy, an animal is unable to move, to digest its food, to reproduce, to grow, or even to breathe. Energy requirement and balance are more important in food-producing animals with their need to synthesize nutrients (e.g., proteins, fat) for deposition into muscle, milk, and eggs. Carbohydrates are the major energy source in the diet of farm animals.
Forms of Energy
Hydrogen plays a prominent role in energy metabolism. During the catabolism of glucose (C6H12O6) by the animal, hydrogen is transferred from glucose to hydrogen receptors, such as nicotinamide adenine dinucleotide (NAD+) and flavin adenine dinucleotide (FAD). These hydrogen acceptors (reducing equivalents) are oxidized in the reactions of the respiratory chain inside the mitochondria to release energy. In biological systems, oxidation of hydrogen is coupled with the synthesis of adenosine triphosphate (ATP). ATP is the readily available form of energy (“molecular energy currency unit”) in the cell. ATP has three components: a nitrogenous base (adenine), the sugar ribose, and the triphosphate (figure 5.1). Energy is stored within the PO4 bonds, and the release of each phosphate bond generates eight kcal of energy.
Forms of Energy
- 1 mole ATP = 8 kcal/mol
- Reducing equivalents
- 1 mole NAD, NADH = 3 ATP
- 1 mole FAD, FADH = 2 ATP

The Fate of Absorbed Glucose and Metabolic Conversions in the Animal Body
Absorbed glucose could be utilized by the animal for several functions. The first priority is the formation of glycogen in the liver, which is stored in muscle and hepatic tissue. However, the body stores very little as glycogen (a starch-like compound), and glycogen could be rapidly hydrolyzed back to glucose through a process called glycogenolysis. This helps in maintaining blood glucose at a narrow range in normal healthy animals. The second priority is oxidation to form energy (a major function) and is described in the next sections. Since glycogen storage is limited, excess glucose is converted to fat and is stored in adipose tissue. This is accomplished by the breakdown of glucose to pyruvate through a process called glycolysis, which is then available for fat synthesis.
The Fate of Absorbed Glucose
- Storage as glycogen in liver and muscle (small amount)
- Oxidization for energy
- Fat synthesis and storage as fat in adipose tissue
How Cells Derive Energy from Glucose: Metabolic Pathways
Cells use different steps to break down the absorbed glucose to carbon dioxide and water through different enzymatic reactions. The catabolism of glucose occurs in two metabolic pathways: glycolysis and the tricarboxylic acid (TCA; also called citric acid or Kreb’s) cycle.
Glycolysis: Enzymes for glycolysis are located in the cytosol of the cell, and glycolysis occurs in this part of the cell. Glycolysis is the breakdown of 6 C glucose into two 3 C end product pyruvates in aerobic metabolism and lactic acid in anaerobic metabolism. It is a catabolic pathway involving oxidation and yields ATP and NADH (reduced NAD) energy. Glycolysis is the pathway by which other sugars (e.g., fructose, galactose) are catabolized by converting them to intermediates of glycolysis. Fructose can be converted to fructose-6-phosphate by hexokinase. Galactose can enter glycolysis by being converted to galactose-1-phosphate followed by conversion (ultimately) to glucose-1-phosphate and subsequently to glucose-6-phosphate (G6P), which is a glycolysis intermediate.
Energy Production Process through Glycolysis: Glycolysis has two phases: an energy investment phase requiring the input of ATP (preparatory phase) and an energy realization phase (pay off) where ATP is made (Figure 5.2). Cells that utilize glucose have an enzyme called hexokinases, which use ATP to phosphorylate the glucose (attaches a phosphorus group) and changes it into G6P. At this point, the cellular “machinery” can begin to process the glucose. Briefly, in the first reaction of glycolysis, hexokinase catalyzes the transfer of phosphate to glucose from ATP, forming glucose-6-phosphate. Thus this step uses ATP, which provides the energy necessary for the reaction to proceed. Glucose-6-phosphate is converted to fructose-6-phosphate and subsequently to fructose-1,6-biphosphate, which is cleaved to dihydroxy acetone phosphate (DHAP) and glyceraldehyde-3-phosphate (G3P). During this process, an additional ATP is required to phosphorylate the intermediate fructose-6-phosphate. Therefore, the “preparation” of glucose results in two molecules of ATP being used for every glucose molecule processed.
During the payoff phase, G3P is further processed to produce pyruvate. During this phase, one NADH and two ATP are produced during the intermediate steps. The DHAP produced can be simply converted into G3P and processed in a similar manner as the first G3P. Therefore, one glucose molecule will result in the production of two NADH, four ATP, and two pyruvate molecules.
Glycolysis: Net Gain of ATP
- Input = 2 ATP
- Produces = 4 ATP and 2 NADH
- Net gain = 8 ATP (aerobic)
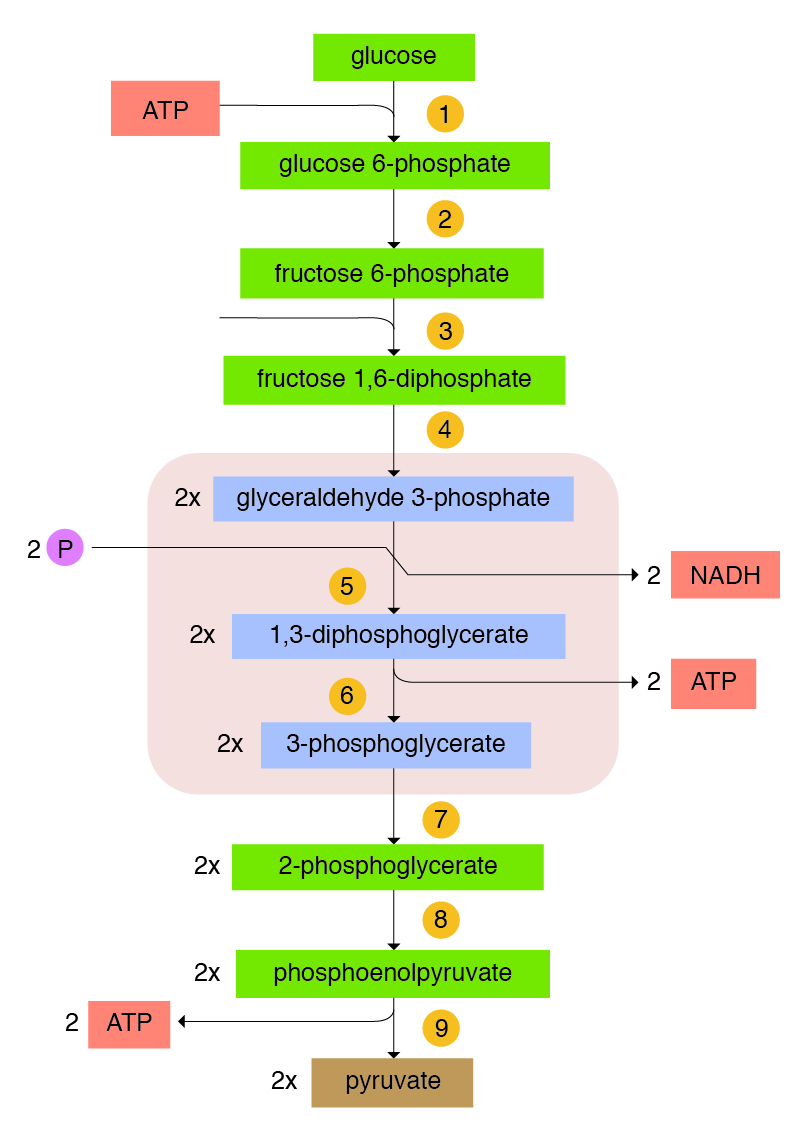
Input: = 1 glucose molecule
Requires = − 2 ATP (Activation)
Produces:
+ 2 pyruvate molecules
+ 4 ATP
+ 2 NADH (= 6 ATP)
Total = 6 + 4 =1 0 ATP
Net gain =10 − 2 = 8 ATP
Glycolysis: Overall Functions
ATP Production:
For each molecule of glucose, 2 ATP (preparatory phase) were used and 2 NADH, 4 ATP, and 2 pyruvate molecules (payoff phase) were generated; which equals a net production of 2 NADH, 2 ATP, and 2 pyruvate molecules, and the net gain of ATP is 8 per mole of glucose.
Production of Other Intermediates:
Glycolysis provides pyruvate for the TCA cycle, amino acid synthesis through transamination, glucose-6-phosphate (glycogen synthesis), nicotinamide adenine dinucleotide phosphate, (NADPH) (fatty acid synthesis; triglyceride synthesis), and dihydroxyacetone phosphate for glycerol synthesis (the backbone of fat).
Fates of Pyruvate in the Animal Body
It is important to discuss the fate of pyruvate generated through glycolysis. Pyruvate has different fates, depending on the conditions of the animal and the cell type.
Fates of Pyruvate
- Lactic acid production
- Acetyl CoA production
Lactic Acid Production: When oxygen is present, there is plenty of NAD+, so aerobic cells convert pyruvate to acetyl coenzyme A (CoA) for oxidation in the citric acid cycle. When oxygen is absent, NAD+ levels can go down, so to prevent that from happening, lactate dehydrogenase uses NADH and pyruvate is converted to either lactate (animals) or ethanol (bacteria/yeast). Anaerobic conversion of NADH to NAD+ provides much less ATP energy to cells than when oxygen is present. Anaerobic metabolism of glucose generates only two ATP per glucose. Once oxygen is depleted for the cell, another system will convert the lactic acid back to pyruvate and produce glucose.
Acetyl CoA Production:
Acetyl CoA Production: Acetyl CoA production occurs in the aerobic state and serves as the main precursor for the TCA cycle, lipogenesis, and ketogenesis (during negative balance). Acetyl CoA is converted to ATP through different steps in the TCA cycle. During this conversion, the enzyme pyruvate dehydrogenase and different B vitamin–containing coenzymes (thiamine, riboflavin, niacin, pantothenic acid) function through a series of condensation, isomerization, and dehydrogenation reactions and produces several different intermediates that are used for fat or amino acid synthesis.
To generate more energy from the glucose molecule, further biochemical processes occur within the animal body. These include the enzymatic step pyruvate dehydrogenase (PDH), which connects glycolysis (cytosol) with the TCA cycle in the mitochondria. During this step, 3 C pyruvate is converted to an active form of acetic acid called acetyl CoA, and CO2 is produced.
Pyruvic acid is decarboxylated and the 2 H ions are picked up by NAD+ and thus it provides two mole of NADH for each mole of glucose (net = 6 ATP produced). This enzymatic step needs coenzyme A and its activity is highly regulated by the concentration of acetyl CoA, ATP, and NADH.
TCA, Citric Acid, or Kreb’s Cycle
Functions of the TCA Cycle
- Recover more chemical energy
- Provide metabolic intermediates (e.g., citrate, α-ketoglutarate, oxaloacetate)
TCA cycle, citric acid cycle, or Kreb’s cycle (named after Hans Krebs who discovered the pathway in 1937) includes a series of enzyme-catalyzed chemical reactions that occur in the matrix of the mitochondria. Several B vitamin–containing enzymes function as coenzymes in the pathway (e.g., thiamine, riboflavin, niacin). The TCA cycle is the key part of aerobic respiration in cells. The TCA cycle also serves as a source of precursors for storage forms of fuels (lipids) and building blocks, such as amino acids, in the animal body.
Prior to entrance into the cycle, pyruvic acid is converted to acetyl CoA through PDH as described previously. Acetyl CoA (2 C) enters the cycle by combining with a 4 C compound called oxaloacetate and forms a 6 C citric acid. This reaction “pulls” the cycle forward. Citric acid undergoes a series (about 10) of enzyme-catalyzed conversions producing different intermediates (e.g., α-ketoglutarate, succinate, fumarate, malate; shown in Figure 5.3).
In many of these steps, high-energy electrons are released to NAD, and NAD molecules also acquire H+ and become NADH. In one of the steps, FAD serves as the electron acceptor, and it acquires H+ and becomes FADH2. In one of the reactions, one ATP is also synthesized from one acetyl CoA. At the end of the cycle, the final product is oxaloacetic acid, which is identical to the oxaloacetic acid that begins the cycle and will pick up another acetyl CoA to begin another turn of the cycle. Altogether, the TCA cycle produces (per one mole of glucose or two moles of pyruvic acid), two ATP molecules, six NADH, and two FADH2. Both NADH and FADH are used in the electron transport chain to generate ATP. Overall, a net gain of 24 ATP per mole of glucose is obtained through the TCA cycle.
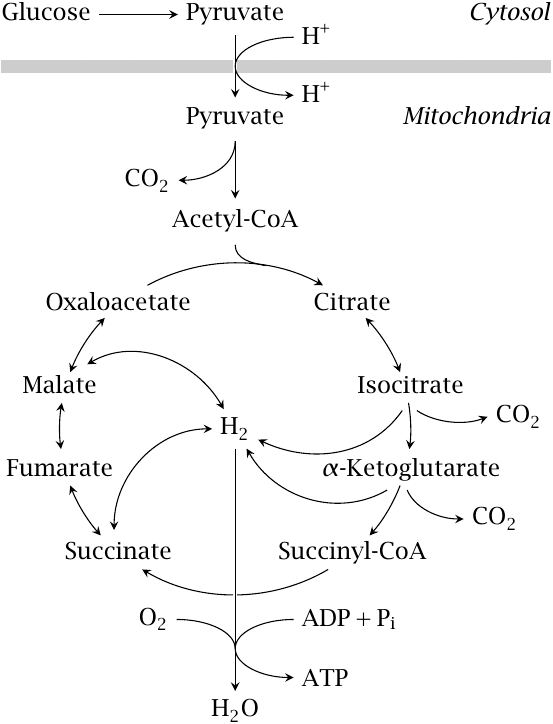
Overall, for every glucose molecule fully metabolized to CO2 and H2O, we receive 38 ATP.
There are eight kcal of energy in every ATP high-energy phosphate bond. Hence net recovery of energy is 38 × 8 = 304 kcal.
The efficiency of converting glucose bond energy into ATP high-energy P bond is therefore 304/674*[1] × 100 = 45%.
The rate-limiting step in the TCA cycle is the combination of oxaloacetate and acetyl CoA to produce citrate. Because of this, it is essential to have adequate quantities of oxaloacetate in order to produce ATP and maintain cell viability. Nutritional diseases such as ketosis result from a deficiency in energy production and therefore require oxaloacetate as precursors to “jump-start” the TCA cycle. The TCA cycle also produces intermediates that serve as a precursor for the synthesis of fatty acids and amino acids. For example, citrate can be used for fatty acid synthesis, while oxaloacetate can be used for nonessential amino acids (glucogenic amino acids or most of the nonessential amino acids, e.g., alanine, serine, cysteine, glycine). During low glucose conditions, these amino acids can produce glucose and α-ketoglutarate can be used for glutamic acid synthesis.
TCA Cycle Intermediates: Roles
- Citrate for fatty acid synthesis
- α-ketoglutarate for glutamic acid (amino acid) synthesis
- Oxaloacetate for non essential amino acid synthesis
Electron Transport System, Respiratory Chain, or Oxidative Phosphorylation: The electron transport chain permits recovery of redox energy associated with NADH and FADH. H ions react with O2 and form water, and thus the electron transport system serves as a source of metabolic water. Electrons are carried to the electron transport system in the mitochondrial wall by NADH and FADH2. Coenzyme Q accepts a pair of electrons and passes electrons singly to cytochrome C and acts as a “traffic cop” for electrons. Oxygen is the terminal electron acceptor and if oxygen is not available, electrons will not pass through the electron transport system and NADH and FADH2 will not be reoxidized. For these reasons, the citric acid cycle will not run either. This is part of metabolic control. Interruption of electron flow can result in production of reactive oxygen species (free radicals). Cellular enzymes, such as superoxide dismutase and catalase, help deactivate reactive oxygen species.
Ruminant Carbohydrate Metabolism
Volatile fatty acids (VFAs) are produced in large amounts through ruminal fermentation and are of great importance in that they provide greater than 70% of the ruminant’s energy supply. The rumen epithelium performs efficient absorption of VFAs through diffusion through a concentration gradient. As they pass through the epithelium, the different VFAs undergo different degrees of metabolism.
The Fate of Volatile Fatty Acids
- Acetate—precursor to Acetyl CoA (TCA cycle; fat synthesis)
- Propionate—precursor to glucose (glycolysis; milk lactose)
- Butyrate—precursor to Acetyl CoA (TCA cycle)
Acetate and propionate pass through the epithelium largely unchanged, but almost all the butyric acid is metabolized in the epithelium to beta-hydroxybutyric acid, a type of ketone body.
The three major VFAs (acetic, propionic, butyric) absorbed from the rumen have somewhat distinctive metabolic fates:
Acetic acid is utilized minimally in the liver and is oxidized throughout most of the body to generate ATP. Another important use of acetate is as the major source of acetyl CoA, and it enters the TCA cycle. Acetic acid is used for the synthesis of lipids (e.g., milk or body fat). A high-roughage diet favors the production of acetic acid.
Propionic acid is almost completely removed from portal blood by the liver. Propionate is converted to succinyl CoA, and it enters the TCA cycle. Within the liver, propionate serves as a major substrate for gluconeogenesis, which is absolutely critical to the ruminant because almost no glucose reaches the small intestine for absorption. For example, in a dairy cow, all the glucose in the milk lactose was synthesized in the liver and most of that synthesis was from propionic acid. A high-concentrate diet favors the production of propionic acid.
For butyric acid, butyrate is split into two acetyl CoAs, and it enters the TCA cycle. Most of the butyric acid that comes out of the rumen as the ketone beta-hydroxybutyric acid which oxidized in many tissues for energy production.
Key Points
- One mole of glucose, if burned in a flame, liberates 674 kcal of heat. Life is equivalent to this process (i.e., respiration is combustion); hence oxidation of glucose in an animal’s body allows for the recovery of the chemical bond energy of glucose in a useable form. Energy is needed for life processes including heart work, protein synthesis, fat synthesis, milk synthesis, or meat and egg production.
- Different metabolic pathways such as glycolysis, tricarboxylic acid (TCA), and the electron transport system, plus one enzyme, pyruvate dehydrogenase (PDH), allow efficient conversion of glucose chemical bond energy into useable energetic intermediates such as ATP.
- Glycolysis yield two net ATP / glucose molecule plus two NADH. Because NADH can be metabolized to ATP and because we get two NADH from each glucose molecule, the net ATP yield per glucose in glycolysis is eight.
- Pyruvate dehydrogenase connects glycolysis with the TCA cycle. The TCA cycle occurs in the mitochondria. PDH generates one NADH and one CO2 for each pyruvate and also generates an acetyl CoA, which is the starting material for the TCA cycle.
- TCA cycle produces two ATP, six NADH, and two FADH2 per each mol of glucose.
- The electron transport within the wall of the mitochondria permits recovery of redox energy associated with nicotinamide adenine dinucleotide and flavin adenine dinucleotide reduced forms (NADH and FADH).
- Other sugars besides glucose are metabolized by these pathways. Fructose may be metabolized into the glycolytic pathways either via fructose-6-phosphate and galactose, a constituent of milk sugar, may be metabolized to glucose-6-phosphate, a glycolytic intermediate.
- For every glucose molecule fully metabolized to CO2 and H2O, we receive 38 ATP. There are eight kcal of energy in every ATP high-energy phosphate bond. Hence the net recovery of energy is 38 × 8 = 304 kcal. The efficiency of converting glucose bond energy into ATP high-energy P bond is therefore 304/674 × 100 = 45%.
- In ruminants, volatile fatty acids (VFAs) serve as a major energy source. Through entry into the TCA cycle, acetate—mainly used for fat synthesis and propionic acid—serves as a major substrate for gluconeogenesis.
- A high-fiber diet favors acetate, and a high-concentrate diet favors propionic acid.
- In dairy cows, milk fat and milk yield are highly affected by the type of carbohydrate fed.
Review Questions
-
-
- What are the three principal fates of glucose after absorption?
- How much ATP is generated during the complete oxidation of glucose under aerobic conditions?
- What is the readily available form of energy for cells?
- The site of glycolysis and the tricarboxylic acid (TCA) cycle in the cell is ______ and ______.
- In ruminants fed concentrate-rich diets, _________ is the major volatile fatty acid (VFA).
- What are the three principal fates of glucose after absorption?
- This VFA is the precursor of glucose in ruminants______________.
- _____________________ links glycolysis with the TCA cycle.
- In ruminants fed roughage-rich diets, _________ is the major VFA.
- A 2 C chemical that enters the TCA cycle to be oxidized to provide energy is __________.
- The end product of glycolysis under aerobic conditions is _____.
- lactic acid
- pyruvic acid
- acetic acid
- citric acid
- Acetyl CoA enters the TCA cycle and condenses with this 4 C compound to form citrate _____.
- malate
- oxaloacetate
- fumarate
- pyruvate
- Complete the following table for total ATP and kcal produced through the three different metabolic pathways for a single glucose molecule under aerobic conditions.
-
Glycolysis | Pyruvate to Acetyl CoA | TCA/Citric Acid | Total ATP | Total kcal/glucose molecule | |
Total ATP |
- *=gross energy of 1 mole of glucose. ↵