Ecological Systems Thinking
To understand systems thinking, we should first align on what a system is. A system is a set of interrelated, interactive, and interdependent cofpomponents. For example, an area of sagebrush steppe is a system of plants, soil, animals, water, solar radiation, and so on (figure 4.1), all of which interact with one another in one or more ways.
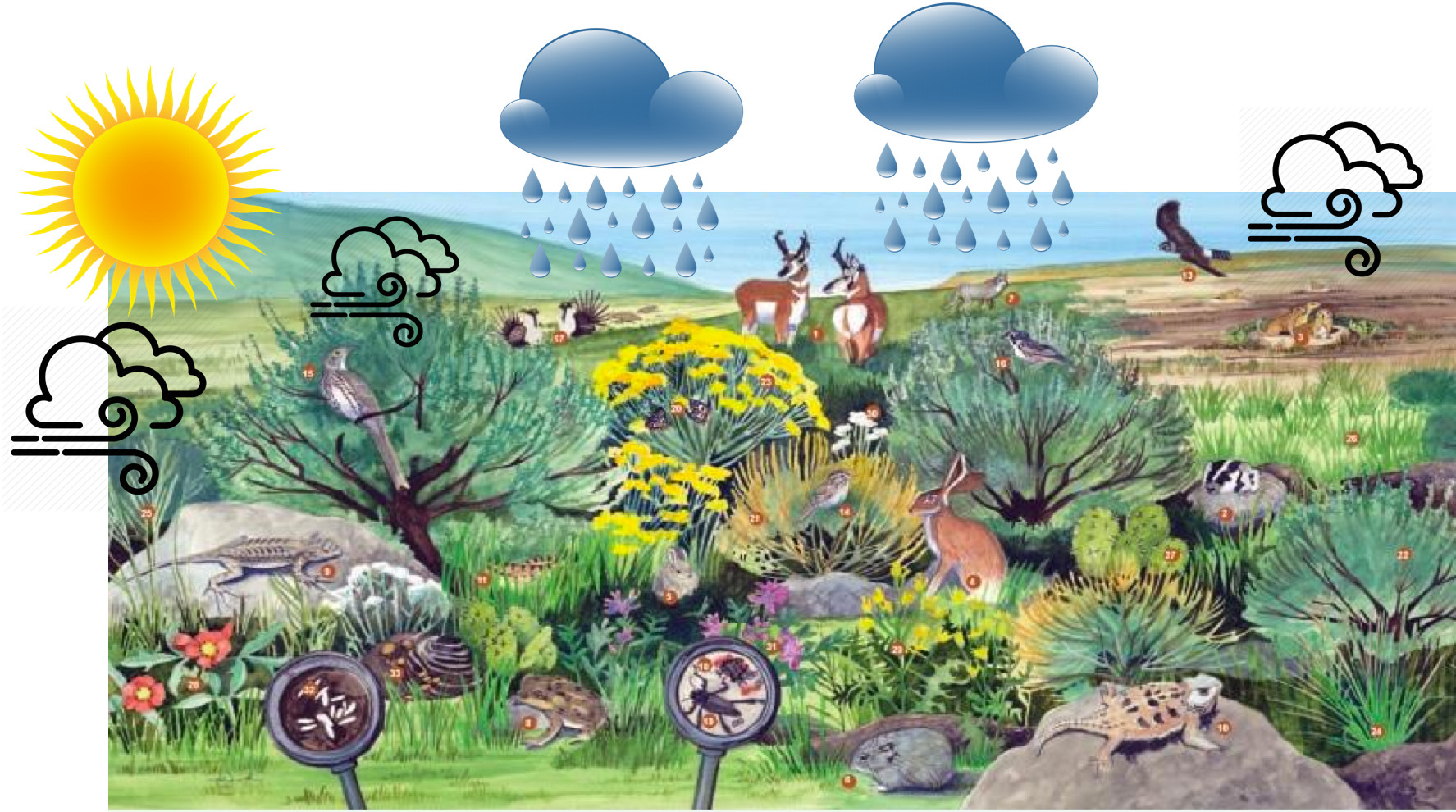
Systems comprise various components, interactions, and controls. Systems have purpose. A good way to think about the purpose of an ecological system is to describe it as an ecosystem providing goods and services. Understanding the fundamental purposes (note plural—more than one purpose) of ecological systems is critical for land use and management.
For a system to function optimally, all components must be present and functional. This characteristic of a system relates to biodiversity and redundancy. When a component, interaction, or control is missing from a system, a ripple effect may be put in motion. For example, when wolves (Canis lupus) were removed from Yellowstone, predation pressure on elk (Cervus canadensis) dropped dramatically, and elk populations increased. Elk prefer to browse on woody species, and as a result of the increased elk population, birch species in riparian areas were overbrowsed; riparian areas, stream channels, and ecosystems degraded. The reintroduction of wolves to the Yellowstone system illustrates the last characteristic of a system: through interactions, controls, and feedback, a system is capable of maintaining stability.
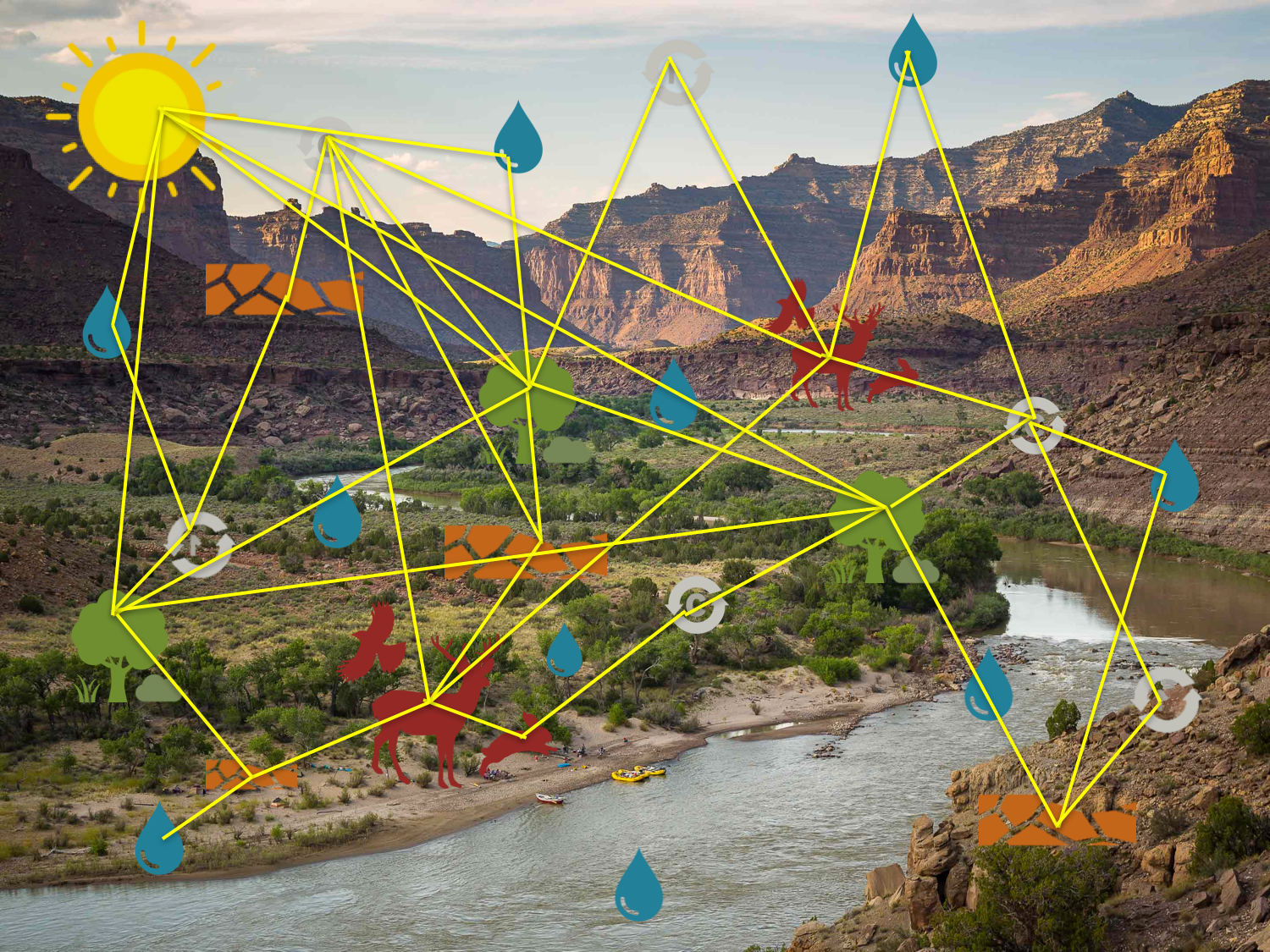
Systems thinking considers the cohesive whole: how all the components relate to and influence one another. Systems thinking reveals how changes in one component, control, or interaction impact other components, controls, or interactions. Understanding these dynamics gives us multidimensional insight into a system. For example, figure 4.2 illustrates many basic connections along the banks of the Green River outside of Moab, Utah.
It is important to remember that ecological systems are highly dynamic and ever-changing. Thinking in systems enables one to better understand system dynamisms and thus to anticipate trajectories of change due to land use, management, or disturbance. What we most readily notice about systems are events, such as a fire disturbance, grazing, or the surface of a landscape view, and these events generally drive our management decisions. What we need to see and understand to make better, holistic, management decisions are the patterns and the system structure that underlie those events.
Section 1: System Components
Components in a system are things that interact, such as plants, animals, water, microbes, nitrogen, and so on. Components may be categorized as abiotic or biotic; components may also be controls. Components each have their own structure function and purpose in the system, all of which must be understood to comprehend the system’s processes, functions, and purposes.
Abiotic Components
Abiotic components are the nonliving elements in the system. They may be single discrete units, such as nitrogen, or an aggregation of many components, such as soil. Looking at figure 4.1, how many abiotic components can you identify? Several are wind, solar radiation, precipitation water, soil, carbon, various forms of nitrogen in the atmosphere and soil, carbon and nitrogen in plants and animals, rocks, and minerals in rocks.
Biotic Components
Biotic components are the system’s living elements. They may be single discrete units, such as a plant, or an aggregation of many components, such as a community of animals. Looking at figure 4.1, how many biotic components can you identify? Several are pronghorn (Antilocapra Americana), sage grouse (Centrocercus urophasianus), Wyoming big sagebrush (Artemisia tridentata wyomingensis), rabbitbrush (Ericameria nauseosa), soil microbes, Great Basin spadefoot toad (Spea intermontana), western wheatgrass (Pascopyrum smithii), bluebunch wheatgrass (Pseudoroegneria spicata), and many more.
Section 2: Interactions
Interactions in a system include any events involving two or more components. For example, the interaction among solar radiation, water, and carbon dioxide is the interaction of photosynthesis. The interaction between a jack rabbit (Lepus californicus) and a coyote (Canis latrans) is one of prey and predator. Thousands of interactions occur in any given ecological system (some will be covered in chapter 5). Each interaction involves its own chain of events, functions, and purposes, all of which must be understood to fully comprehend the processes, functions, and purposes of the system.
Section 3: Controls
Controls in a system are any component that influences an interaction between other components. Controls can be thought of as inputs. For example, solar radiation is an abiotic component in a system, but it is also a control or input of photosynthesis. Water is another example of an abiotic component that is a control or input in many processes within an ecological community.
Components may be controls or inputs removed two to three degrees or more from the primary interaction output of interest. For example, say we are interested in the distribution and population density of coyotes and foxes. The components of water and solar radiation can be viewed as controls because they control plant growth. Jack rabbits eat grasses and forbs as well as nest in and use sagebrush for cover. Jack rabbits are a food source for coyotes and foxes. In this chain of linkages, water and solar radiation are one control of coyote and fox distribution and population density, since without adequate growth of plants for jack rabbit food and cover, the carrying capacity of jack rabbits is reduced; having fewer jack rabbits reduces the carrying capacity of coyote and foxes or shifts their predation targets to other species. Often components as controls are complex interaction and feedback chains.
Section 4: Feedbacks
Feedbacks in a system are outputs that are then routed back into the system to cause an amplifying (positive) or stabilizing (negative) effect.
Positive Feedbacks
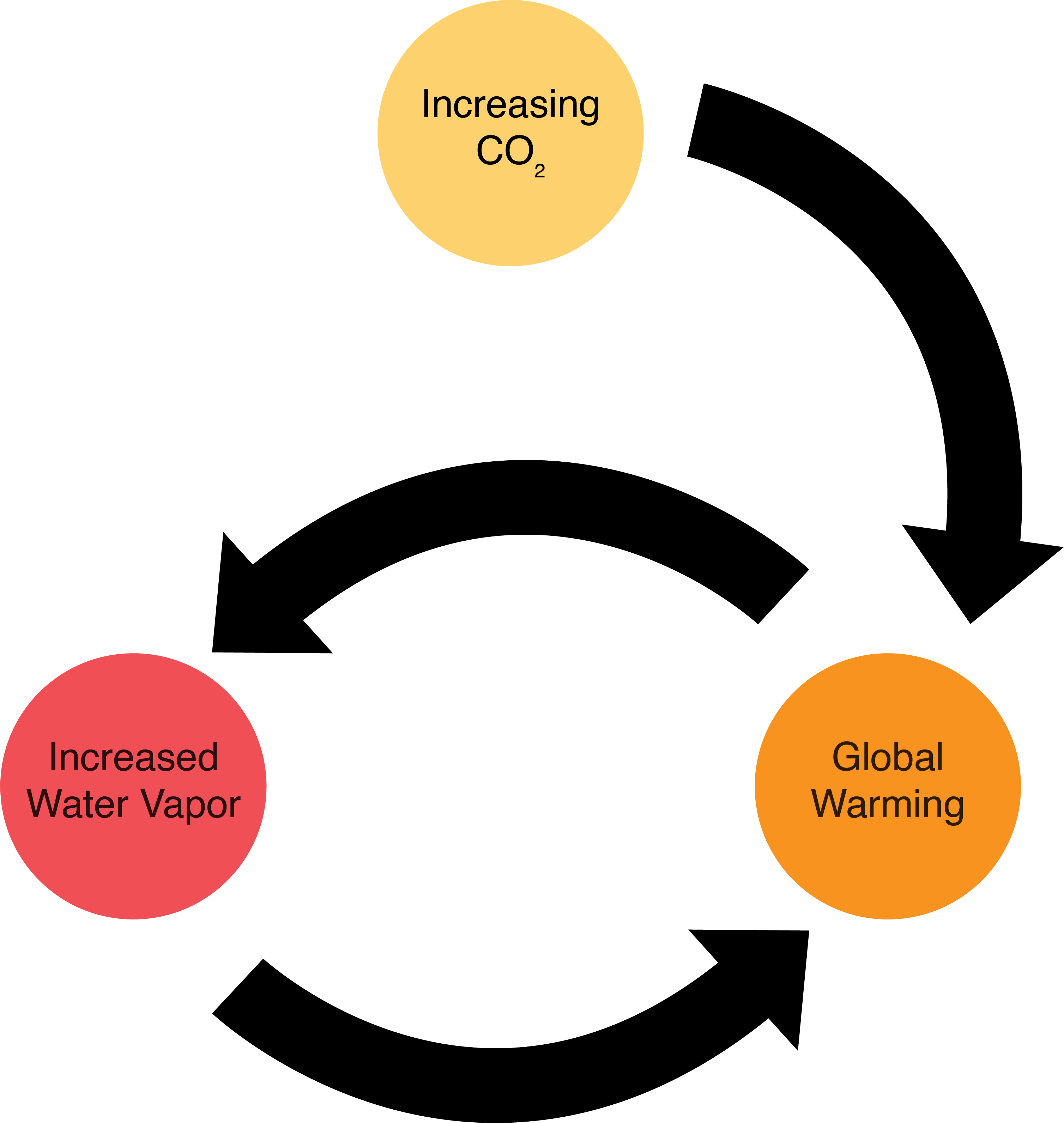
Positive feedbacks in a system cause an amplifying effect, that is, they cause the system to continue to change in the same direction. For example, the more fossil fuels burned, the more atmospheric buildup of CO2 and the greater the greenhouse effect; the greater the greenhouse effect, the warmer the atmosphere; the warmer the atmosphere, the greater the level of evaporation, which further warms the atmosphere, amplifying the ongoing change. (See figure 4.3.)
Negative Feedbacks
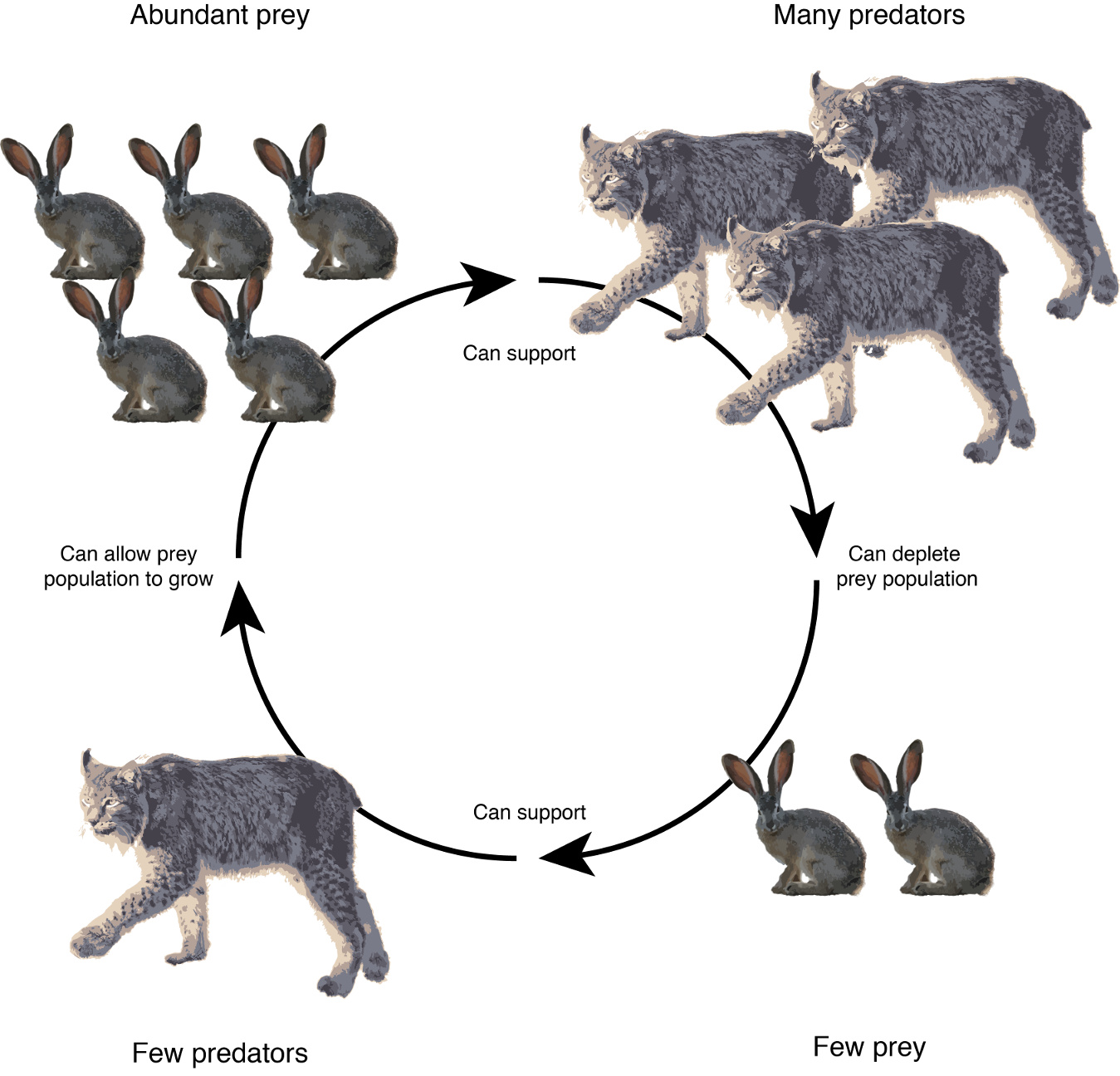
Negative feedbacks in a system cause a stabilizing effect; the input and outputs of the system work toward equilibrium or balance. For example, an abundant hare population can support an abundant lynx population, but as the lynx predate on the hare, reducing the hare population, the lynx population subsequently declines. With a reduced predation threat, the hare population increases, and the cycle starts all over again.
It is important to recognize that feedbacks may have short or longtime frames and that positive feedbacks often lead to degradation. A common positive feedback example involves undisturbed plant biomass production, which leads to more soil organic matter. Sounds like a good thing, right? Consider an undisturbed grassland prairie—lush fields of grass grow year after year, providing habitat for a range of ground-nesting birds and small mammals. As time passes, biomass production and soil fertility increase, but the thatch layer builds up, shading out germinating plants, and high plant density increases competition. Species able to germinate early and grow fast begin to dominate, reducing biodiversity in the system and establishing an incipient monoculture or opening niches for invasive species. So what originally sounded like a good positive feedback over time may not be. All natural systems seek balance.
Section 5: System Patterns and Structure
Patterns and structure, the last characteristics of systems covered in this chapter, can illuminate the broader functions and processes in a system. Understanding interactions generally requires focusing on one or even several components, but understanding patterns and structure requires looking across the system as a whole.
System Patterns
System patterns are the trends, the recurring events. For example, in a system dominated by C4 plants, we can expect late spring germination with a growth season climax in mid- to late summer. In a mountain system adjacent to lower valley or mesa areas, we can expect elk to descend from the mountains after the first heavy snow to graze and browse where vegetation is still accessible. If wildfire burns through a sagebrush system, regrowth is likely to follow a predictable successional trajectory.
System Structure
System structure is the way the components are arranged. In ecological systems, the structure might include the composition, density, and distribution of vegetation communities and the density and distribution of wildlife niches. It could also involve the below-to-above-ground vertical structure of a specific area. Systems structure can mean a trophic cascade, the food web, or any recurring interactions and feedback loops in a system.
We haven’t accounted here for the roles of economics, culture, and society in systems thinking. These aspects are covered in other courses. Layering in these categories of components (e.g., people), interactions (e.g., land use), and controls (e.g., money and values) illuminate even greater and more powerful details of the system. Systems thinking is at the foundation of understanding community ecology and the effective and sustainable management of any ecosystem.