Ecosystem Biogeography
Have you ever wondered why deserts are geographically located in one area and forests or grasslands in another?
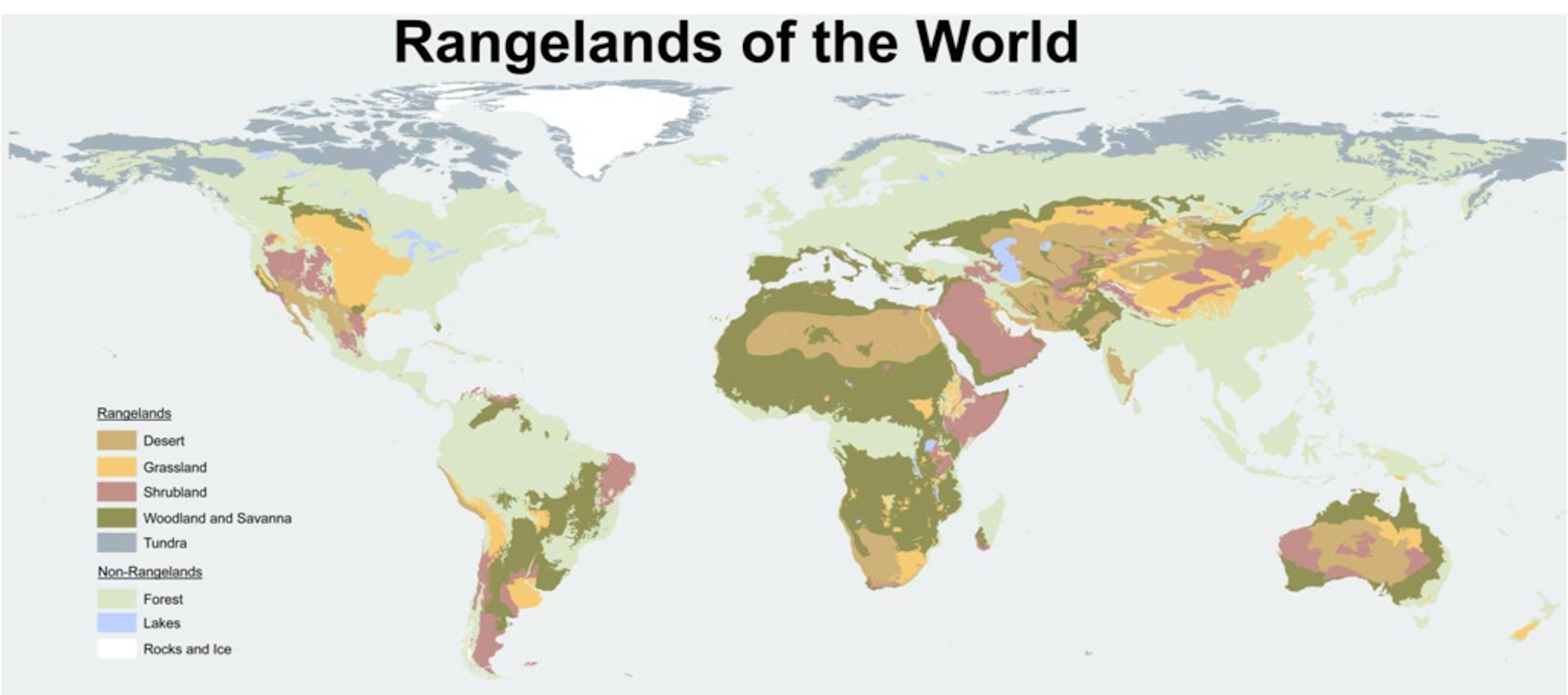
Have you noticed that vegetation changes as you drive from a valley up a mountain and over to the other side?
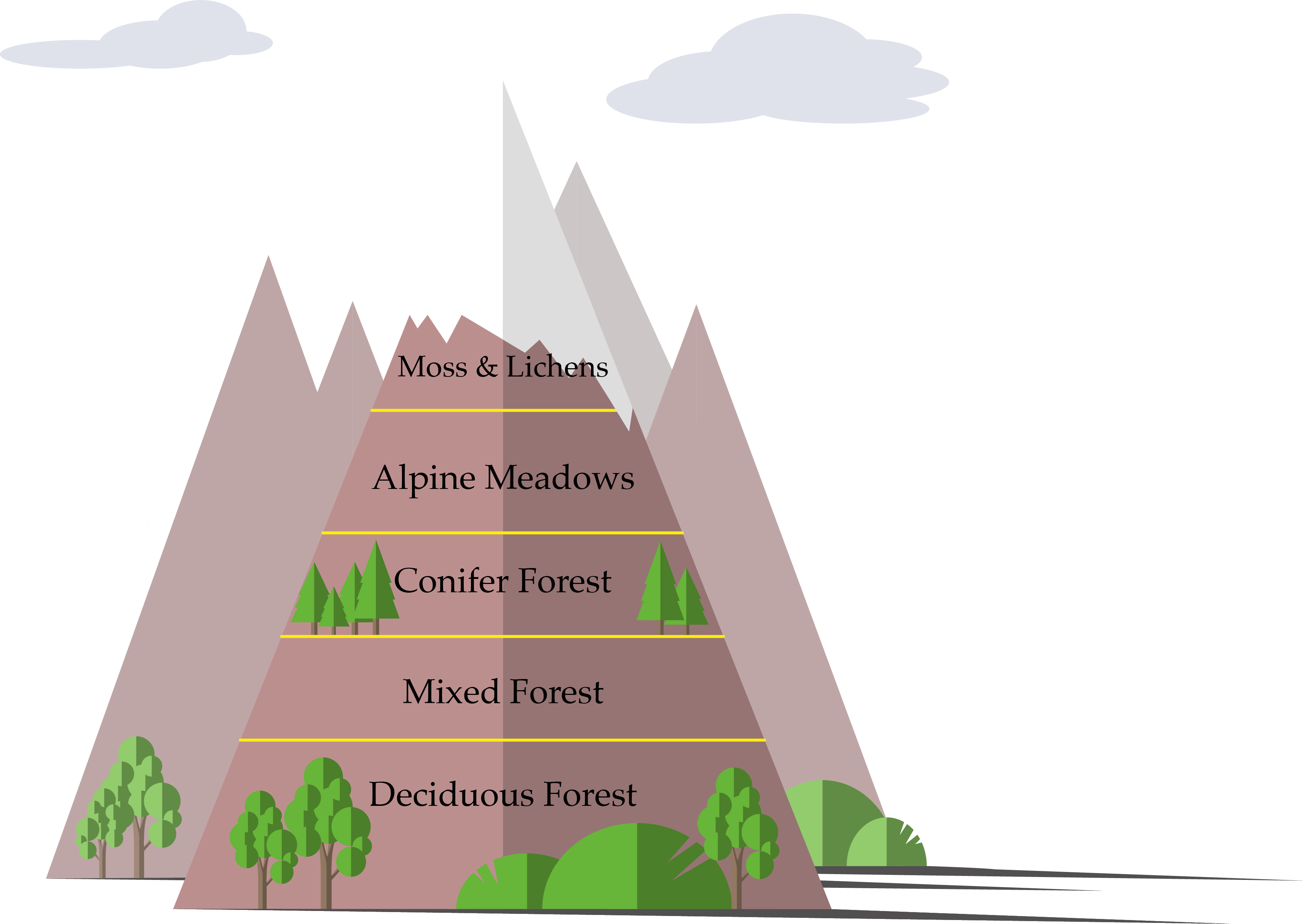
To answer these questions we need to develop an understanding of biogeography that is rooted (no pun intended) in four basic factors: solar radiation, climate, water, and soil.
In this chapter, we will look at how each factor influences the geographic distribution of ecosystems and the structure and function of those systems.
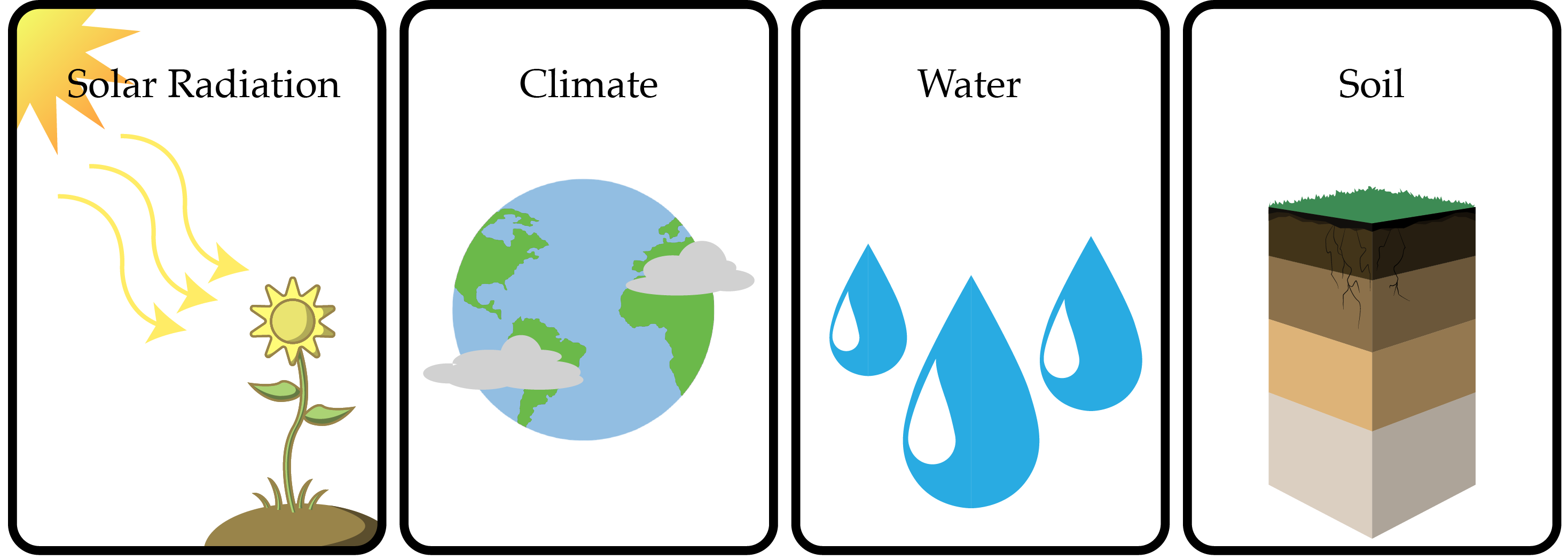
Section 1: Solar Radiation
Solar radiation is the energy radiated from the sun that reaches the Earth’s atmosphere and surface. In one way or another, solar radiation is the origin of all energy on Earth. It is captured by plants and by a variety of technologies and then converted into forms of energy useful for living organisms. However, solar radiation is not evenly distributed across the Earth’s surface: the amount of available energy varies around the globe. This uneven distribution of solar radiation underlies not only the distribution of ecosystems but also the distribution of climate, soil types, species, and land uses.
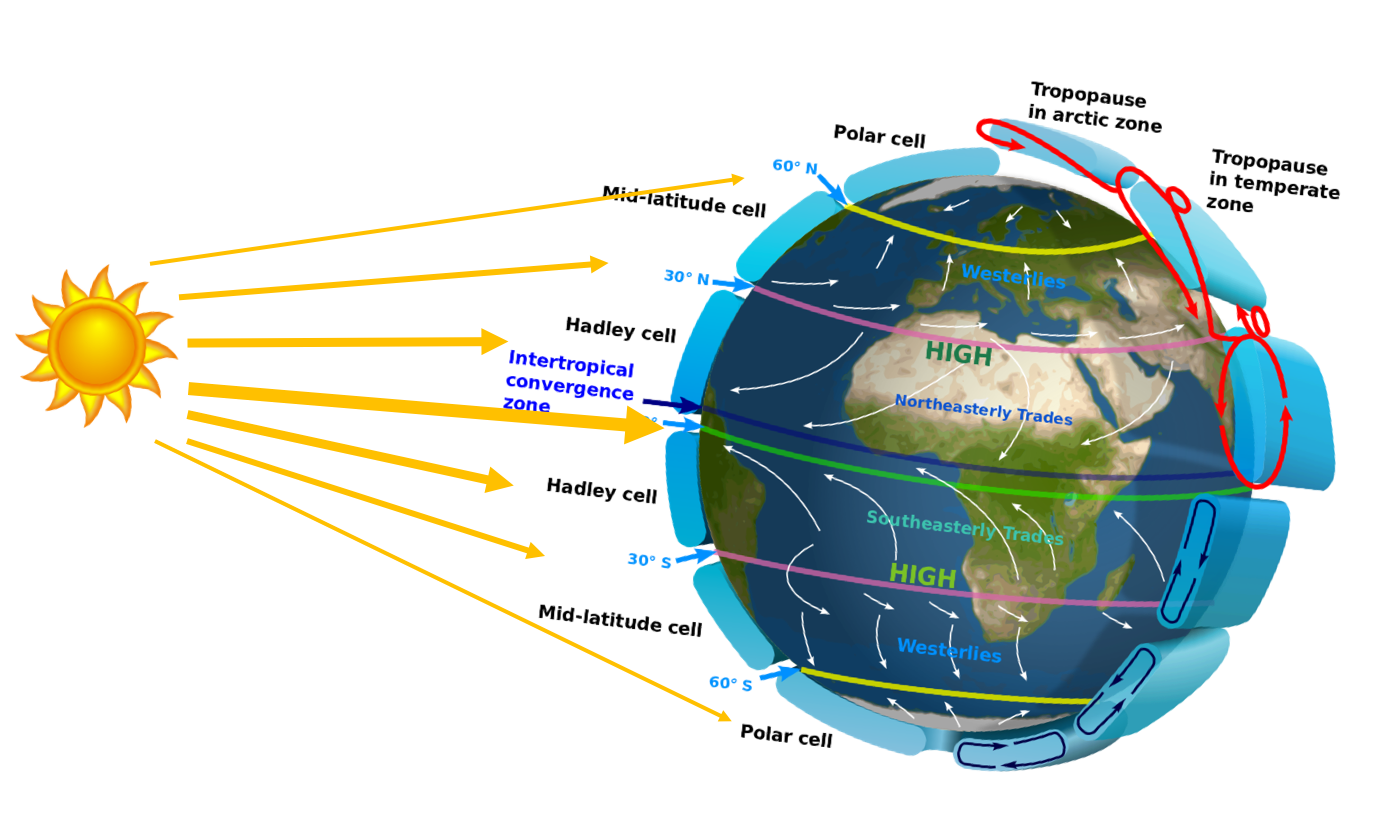
The distribution of solar radiation across the globe is influenced by a number of factors:
- Distance from the sun
- Declination of the sun
- Latitude
- Slop
- Length of day
- Time of day
- Atmospheric conditions
Distribution of Solar Radiation: Distance from the Sun and Latitude
The Earth rotates on a tilted axis, and therefore only the equator is at a direct ninety-degree angle to the sun; the equator thus receives the most solar radiation. Intensity of solar radiation decreases as latitude increases, both north and south, because solar radiation is spread across a greater area.
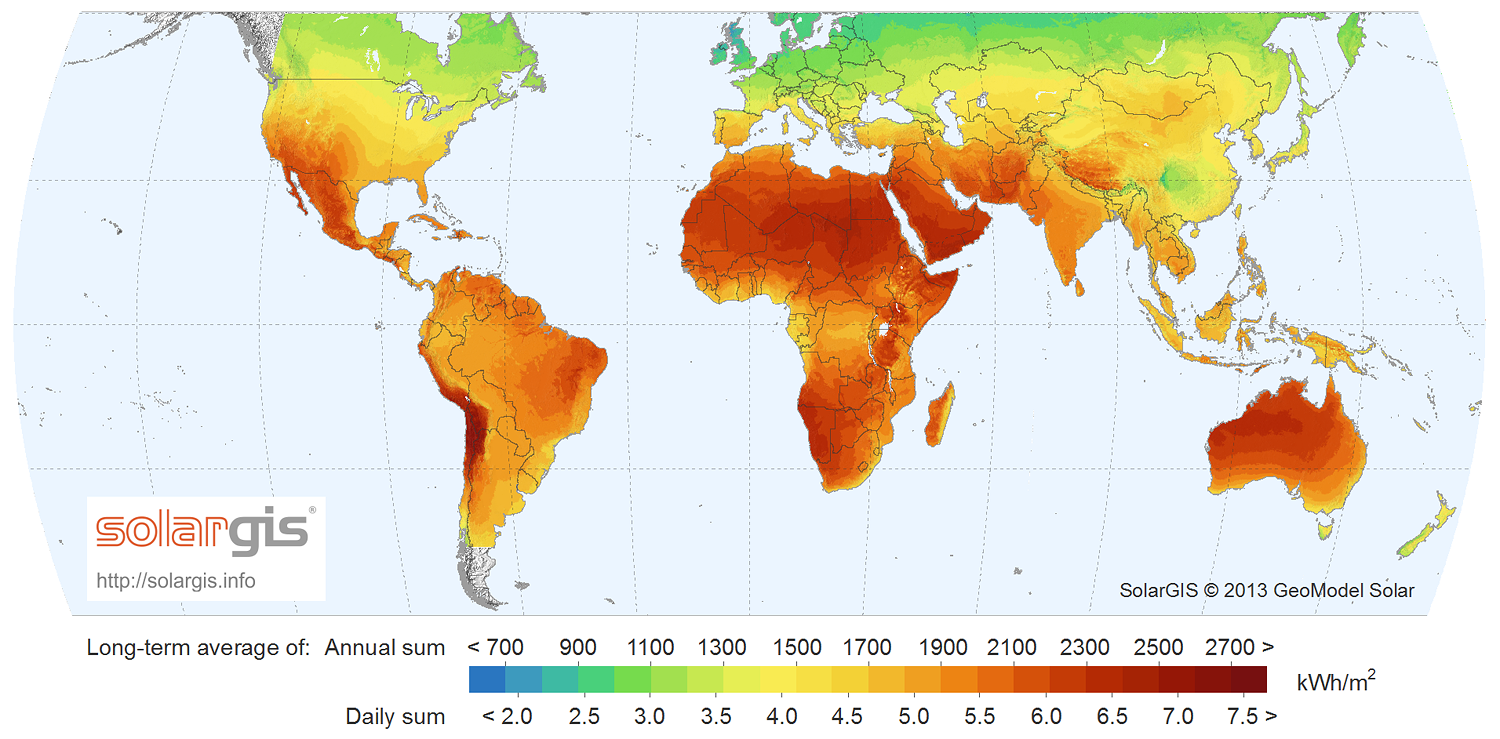
The amount of solar radiation reaching the Earth’s surface at any given time is called insolation and is measured in Wm2 (watts per meter-squared).
Distribution of Solar Radiation: Declination of the Sun
The Earth’s elliptical orbit around the sun and the declination of the Earth (relative position of the sun with respect to the equator) shifts from 23.45 degrees north to 23.45 degrees south throughout a single year. It is this shift in declination that underlies the variations in climate that we refer to as seasons.
Distribution of Solar Radiation: Slope
What does “north face” mean? Yes, it’s a clothing line—one named after the north aspect of a mountain. The north side of any area of elevated topography receives less solar radiation than its other aspects; such areas are therefore colder and tend to have wetter soils with lower densities of soil organisms, less integration of soil organic matter into the soil profile, and more acidity.
Distribution of Solar Radiation: Length and Time of Day
As latitude increases, the length and intensity of the photoperiod (hours of light within the 24-hour period of light and dark) decrease. This variation in photoperiod and intensity is determined by the latitude’s distance from the sun and by the declination. Photoperiod influences both the growing season and the duration of daytime photosynthesis.
Distribution of Solar Radiation: Atmosphere
Atmospheric composition, density, and distribution have a substantial effect on the amount and timing of solar radiation reaching the surface of the Earth. The primary gases in the Earth’s atmosphere (water, oxygen, carbon dioxide, and ozone) all absorb and reflect solar radiation.
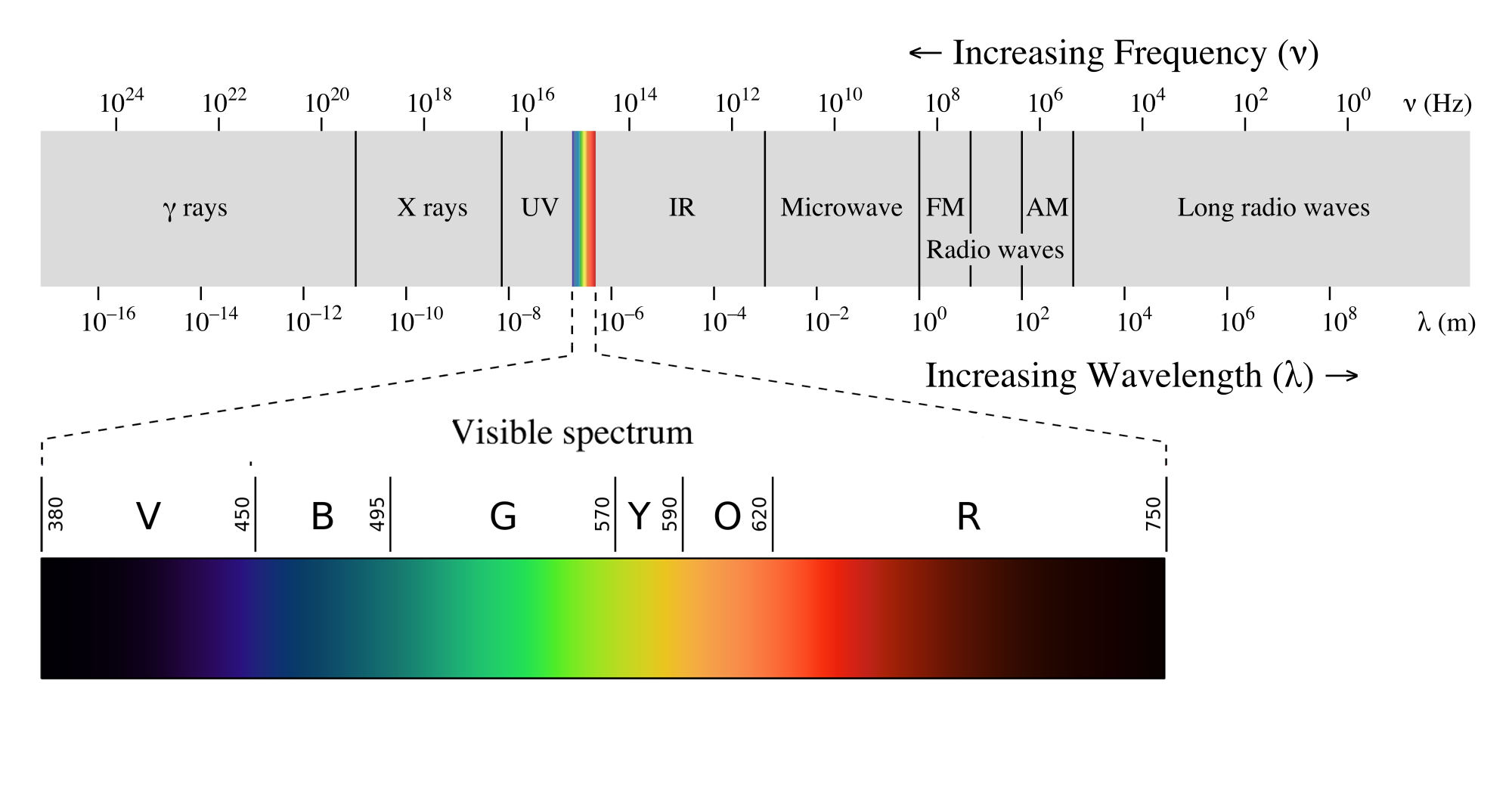
Far red: Elongation of internodes, seed germination, and photoperiod response
Red: Absorbed by chlorophyll for photosynthesis, germination, and photoperiod response
Green: Reflected (it’s why plants are green)
Blue: Absorbed by chlorophyll for photosynthesis
Ultraviolet: Damaging
Section 2: Climate
In a moment we will connect solar radiation to climate, but before we do that, let’s differentiate between weather and climate. Weather is the day-to-day, hour-to-hour state of the atmosphere; it is what meteorologists predict based on atmospheric conditions. Climate consists of patterns of precipitation, temperature, humidity, barometric pressure, and wind over time. For example, a Mediterranean climate is characterized by cool, wet winters and hot, dry summers. Weather is a driving factor in determining the degree of vegetation growth in a given season. Climate is a driving factor in determining biome type.
The primary drivers of climate are solar radiation, the atmosphere surrounding the Earth that holds in or releases solar radiation, and topography. Solar radiation from the sun hitting the Earth’s surface and heating it drives wind patterns and the water cycle, which in turn influence the distribution of weather patterns across the globe.
Precipitation
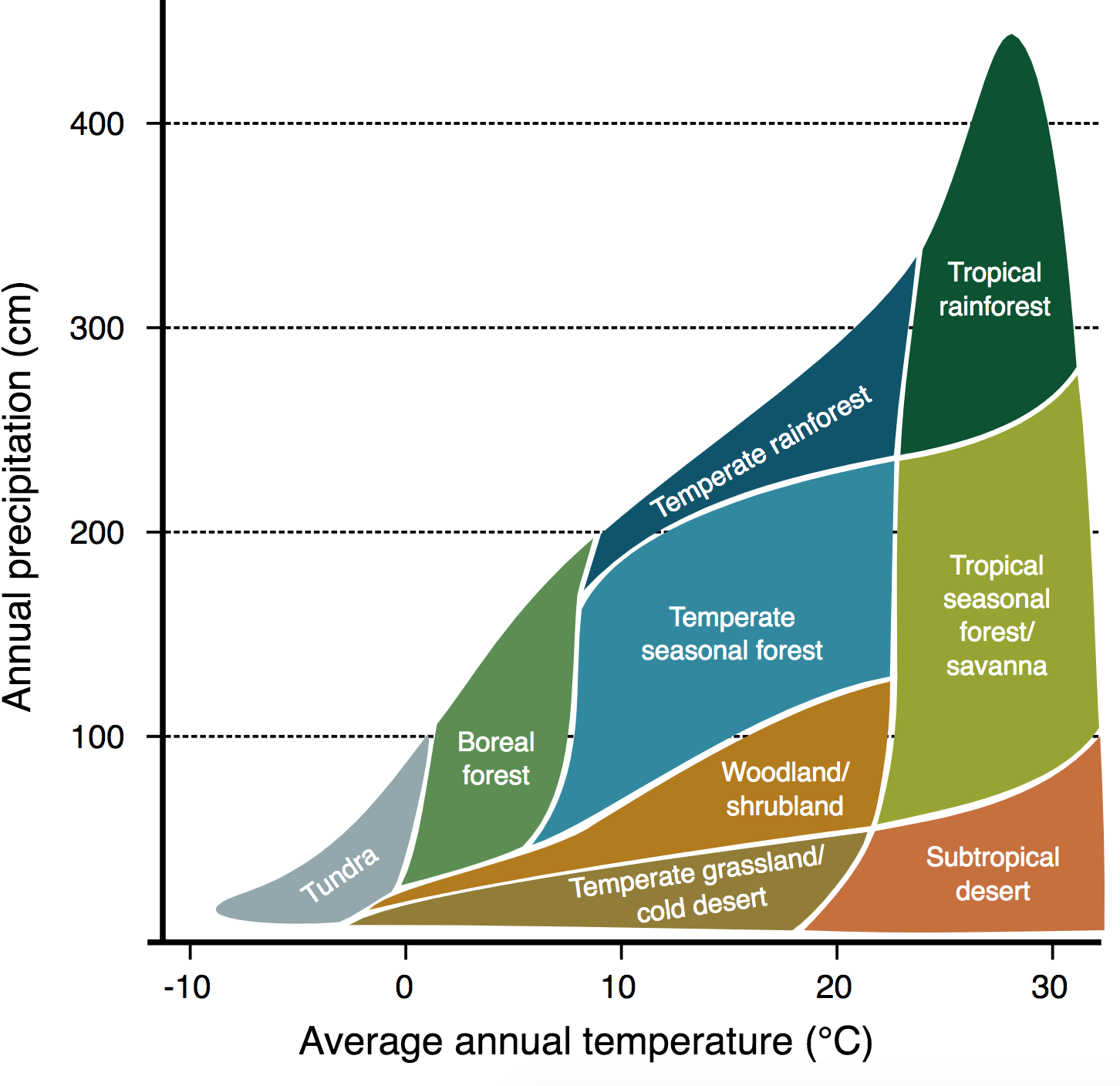
Precipitation is the primary factor determining vegetation production and, concomitantly, ecosystem function and dynamics. For example, the Pacific Northwest has high precipitation and is well known for producing the conifer forests that provide much of the country’s pine and cedar lumber. Most arid and semiarid ecosystems covered in this course have low precipitation and some degree of drought. Referring to Whittaker’s biome graph, we see that deserts, shrublands, and woodland savannas average annual precipitation of less than 100 cm. Although rain is generally the first form of precipitation to come to mind, snow is a significant form of precipitation in some of these ecosystems.
Timing and Intensity of Precipitation: Deserts
Hot deserts in the far western United States generally receive winter precipitation from ocean-borne storms driven by global wind patterns that push moisture inland and up against the southern Sierra Nevada. These storms then track east or northeast across the southwestern United States. These precipitation events generally cover a wide area and are of long duration and low intensity. The desert systems can thus absorb and store the precipitation.
Hot desert areas that border Northern Mexico, the Great Plains, and the Gulf of Mexico receive summer rainfall in the form of monsoons driven by intense summer heating of the Earth’s surface and concomitant large shifts in atmospheric circulation patterns. Cyclonic thunderstorms of short duration and high intensity form in the Gulf of Mexico and track west and northwest. These storm systems generally result in surface water run-off and soil erosion and are immediately followed by high evapotranspiration rates due to high summer temperatures.
Timing and Intensity of Precipitation: Shrublands and Woodlands
Whereas hot desert precipitation is largely driven by the heating of the Earth’s surface and by atmospheric circulation patterns, in northern latitudes precipitation in shrubland and woodland systems is driven by topography, elevation, and geographic location. Further, while desert annual precipitation is primarily in the form of rain, shrubland and woodland systems receive a substantial portion of their annual precipitation in the form of snow.
Other Factors Influencing the Effectiveness of Precipitation
Regardless of the type of system, precipitation infiltration and ground storage are influenced by soil texture, slope, and vegetation cover.
The percentage of sand in soil correlates with its water-holding capacity: the higher the percent of sand, the lower the water-holding capacity. For clay in soil, the higher the percent of clay, the higher the water-holding capacity, to the degree that the ionic bonds in clay soils can limit soil water movement and availability to plants.
The greater the degree of slope, the greater the degree of surface water run-off, although this can be mitigated by the degree of vegetation canopy cover and vegetation basal area and distribution, both of which obstruct surface water flow. Other surface elements, such as gravel and rocks, can perform the same function.
Vegetation canopy intercepts precipitation and channels it to more slowly to the soil surface, increasing infiltration.
Temperature
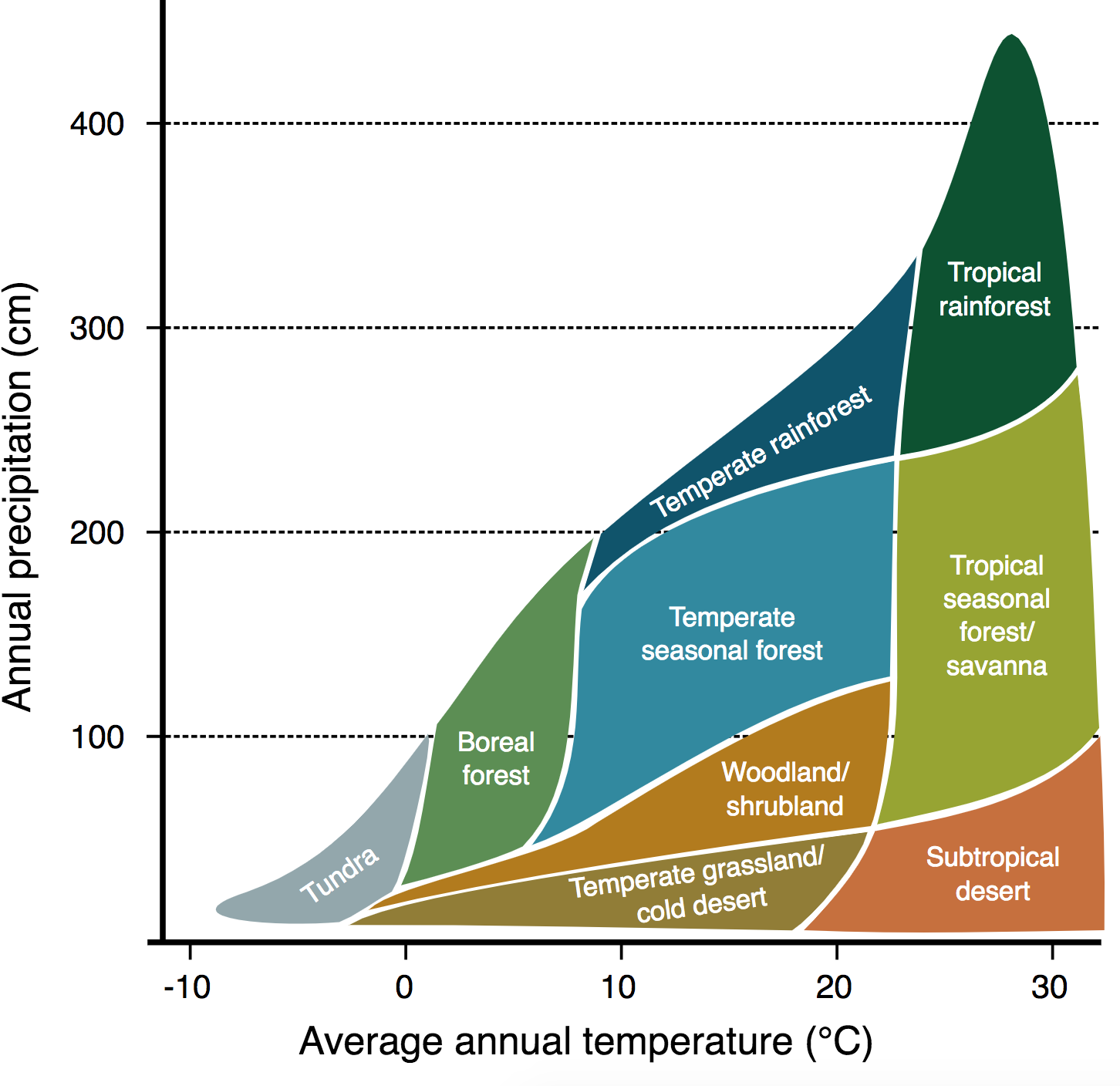
While deserts, shrublands, and woodland savannas all have low average annual precipitation (below 100 cm), the temperature variance is much greater (below 0°C to over 30°C), as these systems span from approximately 30° north to 45° north. Not only is there variation across these systems, but there can also be substantial diurnal temperature variation, the difference between daily minimum and maximum temperatures. This variation in temperature influences evapotranspiration rates, growing degree-days, and likelihood of frost.
Looking across the United States at the average diurnal temperature variance in July (the height of the growing season for most vegetation), we see minimal diurnal temperature variation east of the Rocky Mountains, but from the Rocky Mountains west, particularly in the intermountain region, we see up to a 45.5°F diurnal variation.
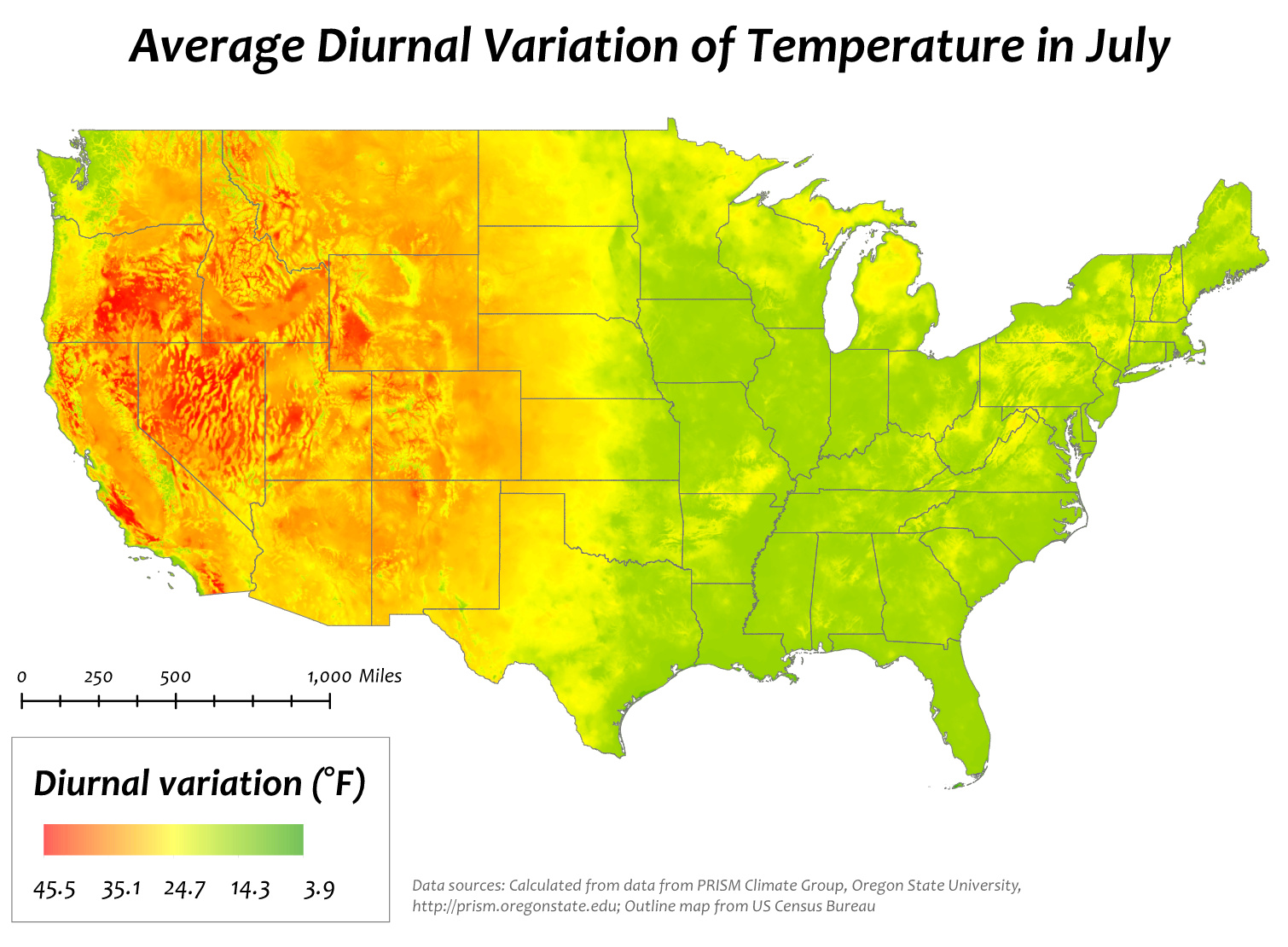
Evapotranspiration
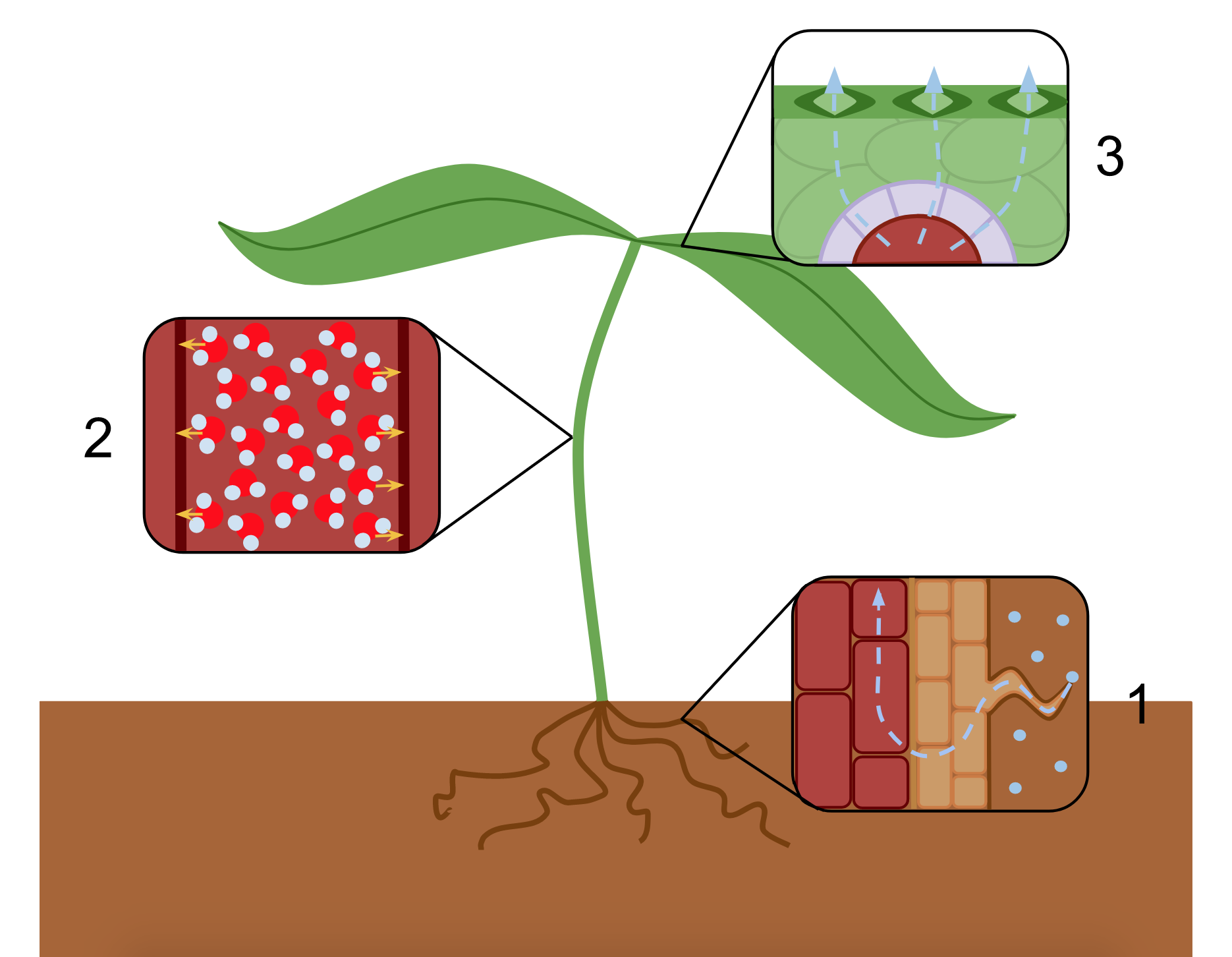
Temperature and wind are primary factors that drive evapotranspiration, the loss of surface and ground water to the atmosphere directly from the surface of the Earth (evaporation) and from plant leaves (transpiration). Recall that plants are key components of the water cycle, as adhesion and cohesion move water from the soil through the roots to the xylem and then throughout the plant, and some of that water is lost as vapor to the atmosphere through stomata on leaves. Evapotranspiration greatly influences water storage and availability in deserts, shrubland, and woodland systems. In these arid and semiarid systems, however, many plant species are adapted to mitigate transpiration. (We will discuss some of these species and their adaptations in chapter 2.)
Growing Degree-Days and the Likelihood of Frost
Each plant species has a specific mean minimum temperature required for growth and development. Growing degree-days are the number of days on which the temperature is at or above a mean minimum temperature. In assessing an ecosystem, close attention is paid to when temperatures facilitate plant growth and development, but equal attention is paid in hot desert systems to periods when freezing temperatures persist for more than twenty-four hours, as a high degree of plant mortality can occur.
Drought
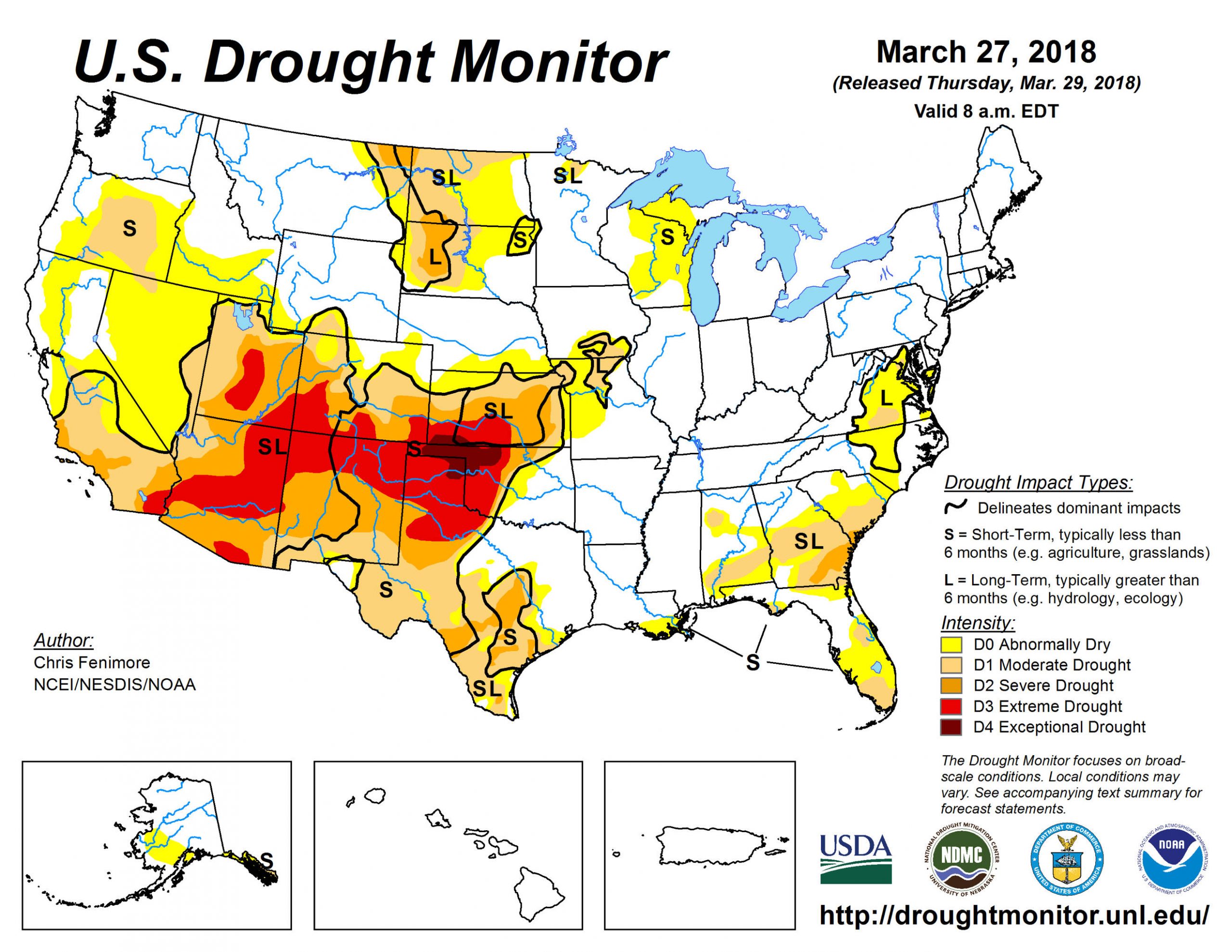
Lack of precipitation and high evapotranspiration causes drought, which can be a persistent issue in these arid and semiarid systems. Drought is the condition in which growing season precipitation falls below 75 percent of the normal annual level. The US Drought Monitor watches precipitation levels very closely and generates daily maps of current and predicted drought.
Understanding the water balance of a system is another lens through which to view drought. If you think about a bank checking account, it has a balance, deposits, and withdrawals. The aim is to cover all the withdrawals and maintain a balance. If we apply this to water in a system it may look like the graph to the right. This type of climate graph is also known as a Walter Climate Graph.
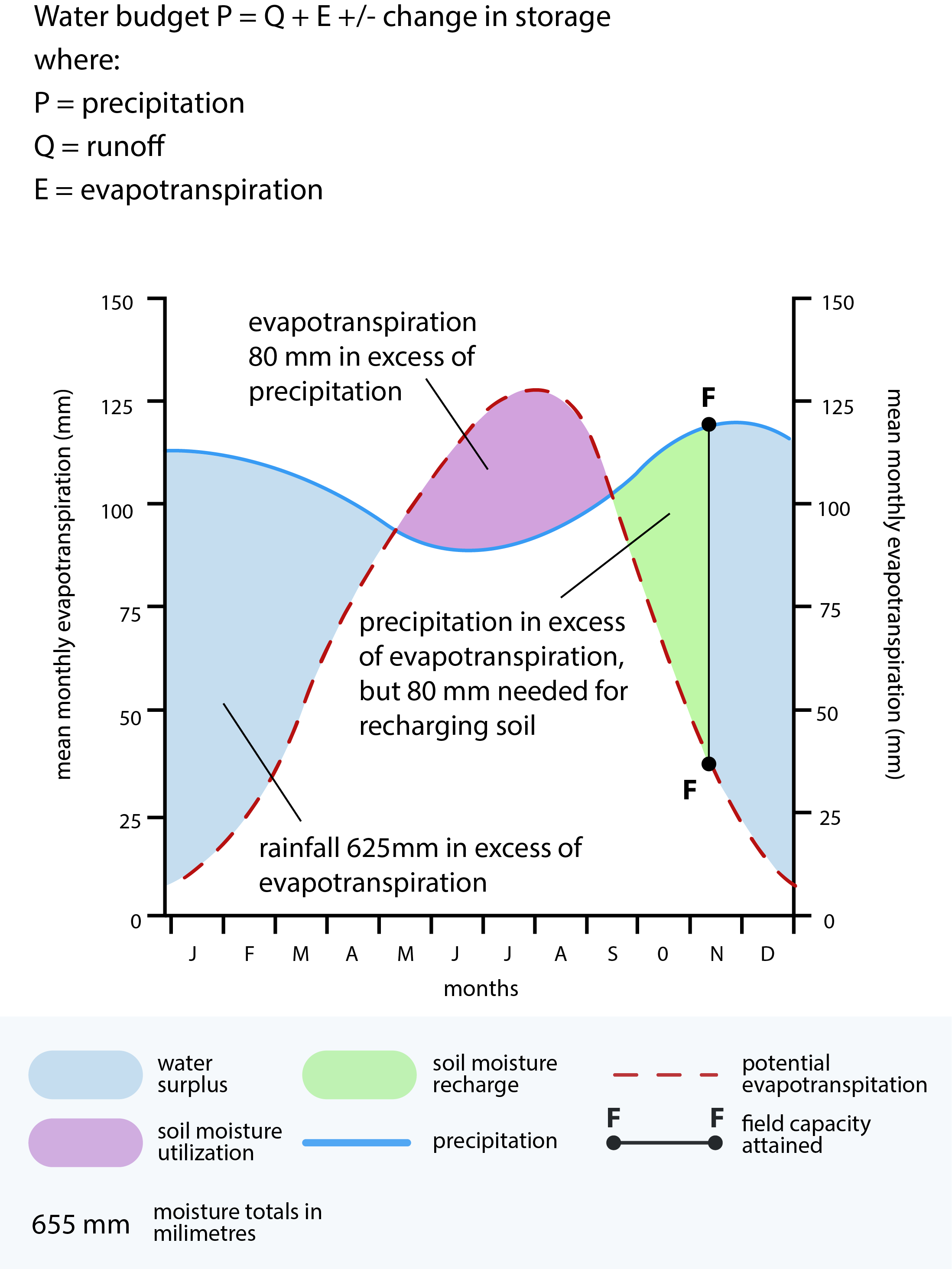
A number of plants in arid and semiarid systems are what are referred to as “drought tolerant”—they compensate for the shortage of water deposits by adjusting their withdrawals. One such adjustment, the ability to reduce transpiration, or evapotranspiration, was noted above. (We will discuss drought-tolerant species in chapter 2.)
Section 3: Topography
Topography influences the two other factors that underlie biogeography: climate and geology. As elevation increases, temperature decreases. As noted in section 2.2.1 above, temperature influences rates of evaporation, growing degree-days, and period of frost, all of which influence the type, species, and abundance of plants in a given area.
Topographic features can also strongly influence the biogeography of the semiarid and arid biomes that are the focus of this text:
- Orographic effect
- Basins and playas
- Alluvial fans and bajadas
Orographic Effect
Looking at a map of precipitation distribution across the United States, we see a narrow strip of high precipitation in the Pacific Northwest while the rest of the western United States has low annual precipitation. This distribution is the result of the orographic effect.
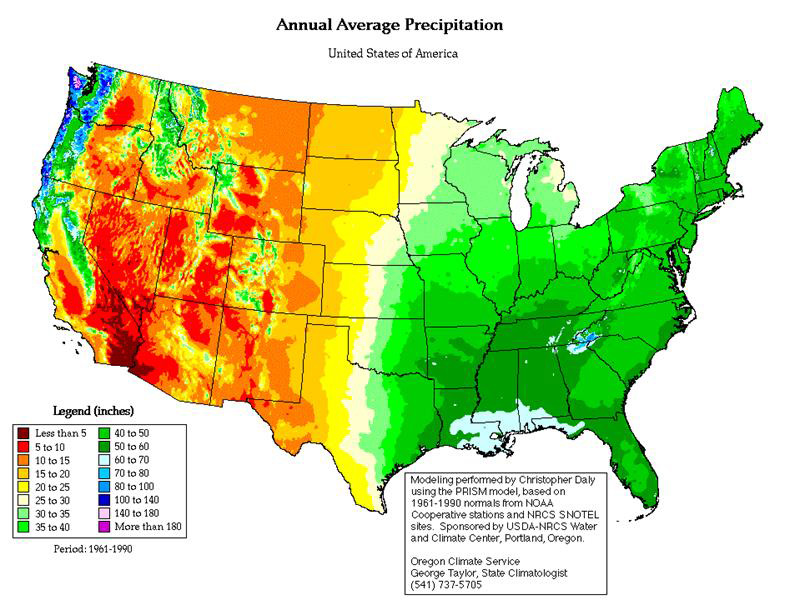
The orographic effect describes what happens when warm air masses flow inland off the ocean and come up against a mountain. As the warm air rises, it cools, and moisture condenses into rain; by the time the air mass goes over the mountain, the water it contained is essentially wrung out. On the other side of the mountain—that is, on its leeward side—the air mass is dry, and precipitation is reduced.
Applying the orographic effect to Oregon—water evaporates off the Pacific Ocean. These water-laden air masses move over land, hit the Oregon Coast Range, and rise, leading to rain over the mountains. The Coast Range isn’t high enough to “wring out” all the water, however, so the air masses continue to rain over the Willamette Valley. As they move through the valley, the water cycles from the air to the land surface and back to the atmosphere until the masses reach the Cascade Range.
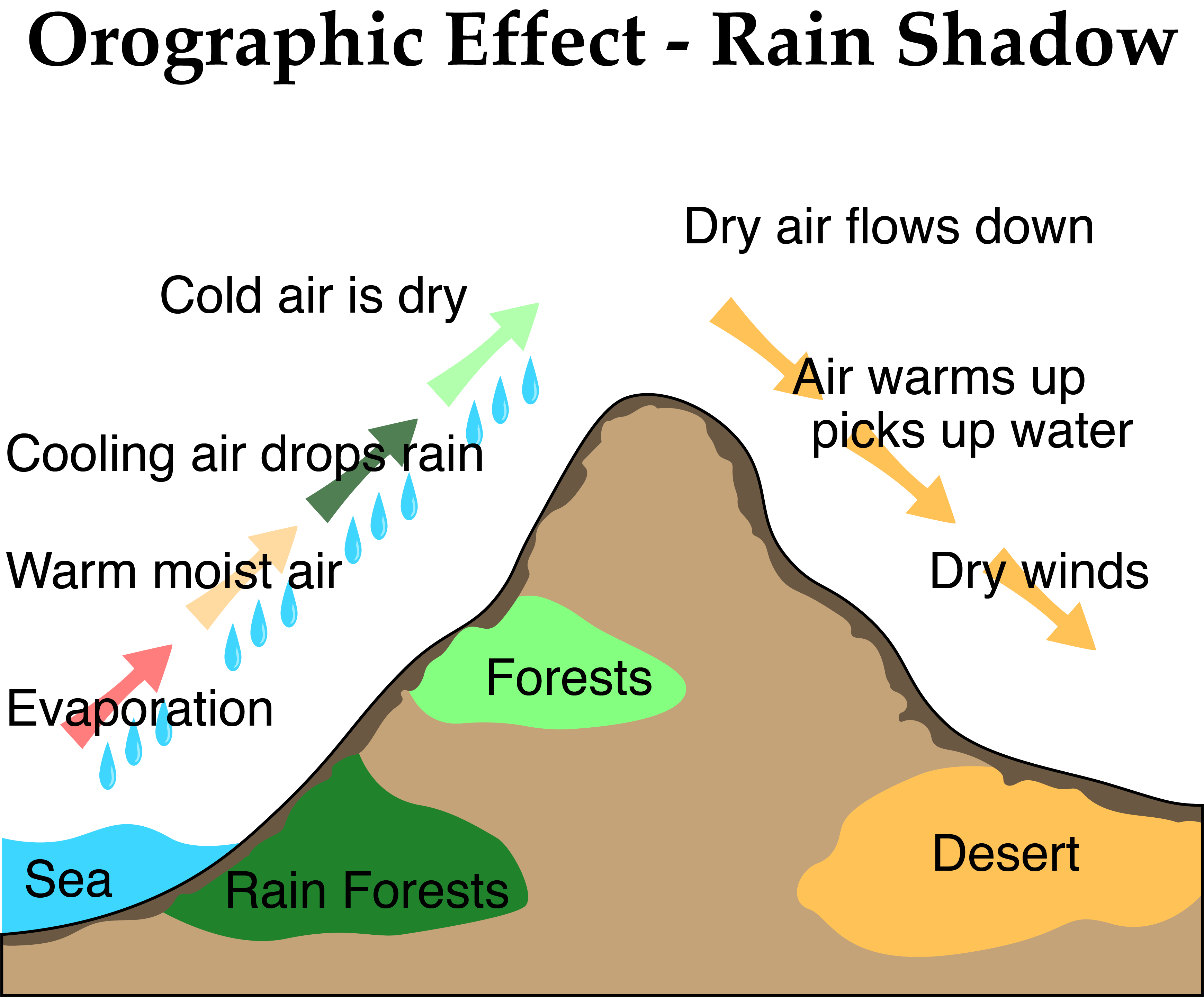
As air masses move up the Cascades, it rains or snows, depending on the season. Because the Cascade Range is much higher in elevation than the Coast Range, much of the moisture is wrung out as the air masses move up the mountains; by the time they have ascended and passed over the mountain, very little moisture remains, and the land east of the Cascades—lying in what is referred to as the “rain shadow”—receives considerably less precipitation than the western side of the mountains.
Driving up and over the Cascades makes the relationship between vegetation and precipitation clear. The western base of the mountain exhibits a lush growth of grasses, forbs, and hardwood trees. As you begin to traverse up the mountain, hardwoods covered in moss slowly transition to fir trees covered in moss, to just fir trees, to fir and pine trees and shrubs with much less dense grasses and forbs . . . to just pine trees and shrubs . . . to shrubs, grass and forb systems around Bend and across central Oregon.
The orographic effect is the primary reason most of the intermountain region of the western United States is semiarid and arid.
Basins and Playas
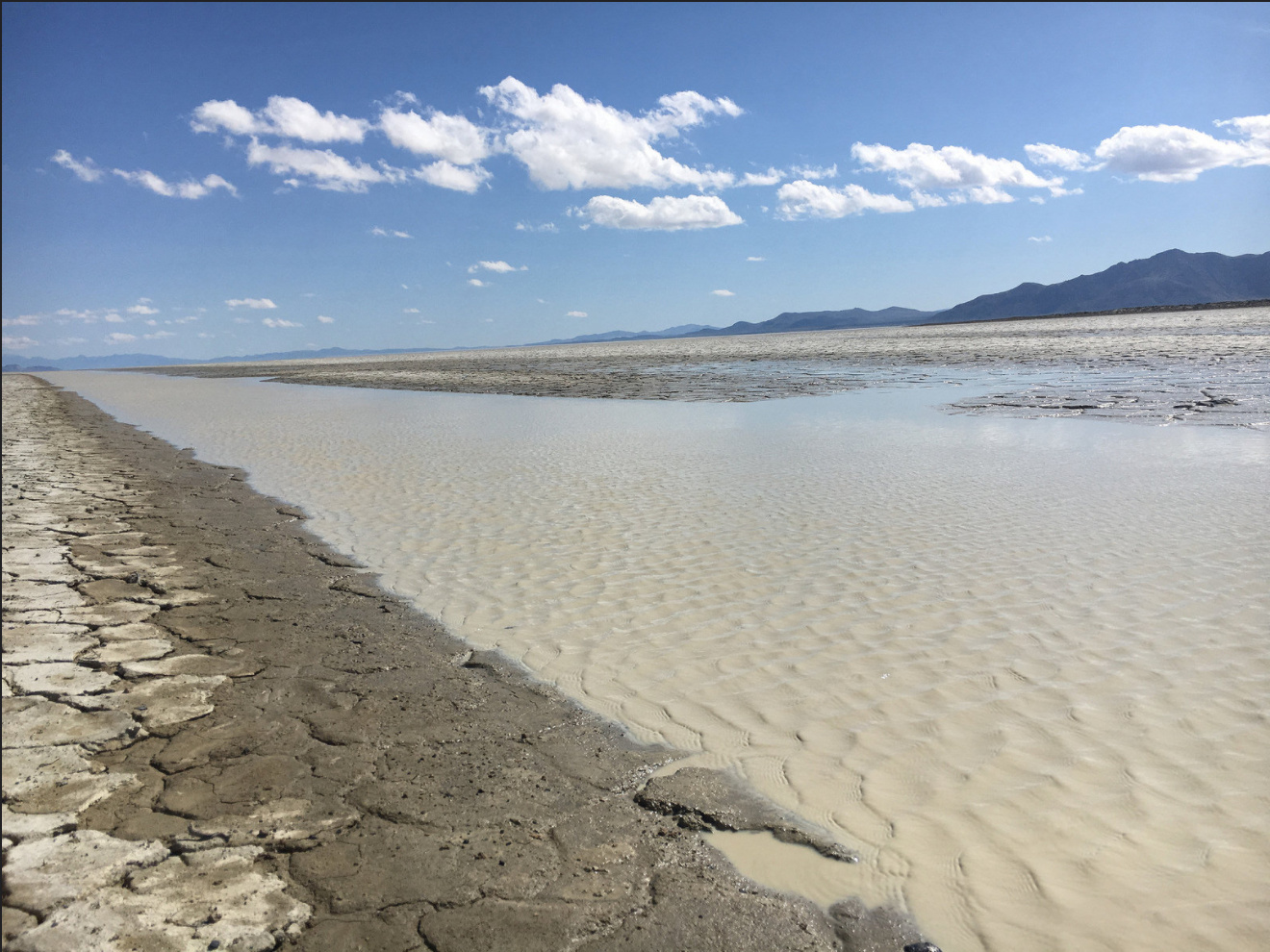
Large areas of the western United States are basins, lower elevation areas flanked by parallel mountain ranges. The Great Basin is flanked by the Cascade and Sierra Nevada Mountains to the west and the Wasatch Range of the Rocky Mountains to the east. This topographic phenomenon is the product of tectonic movement and expansion. A common feature of basins is playas, ephemeral lakes that are formed in land depressions throughout a basin that collect snowmelt and precipitation run-off from surrounding mountains. When the water in the playas evaporates, it leaves deposits high in salt and calcium. The Saltbush Desert of western Nevada and eastern Utah is a product of playas and geologic history.
Alluvial Fans and Bajadas
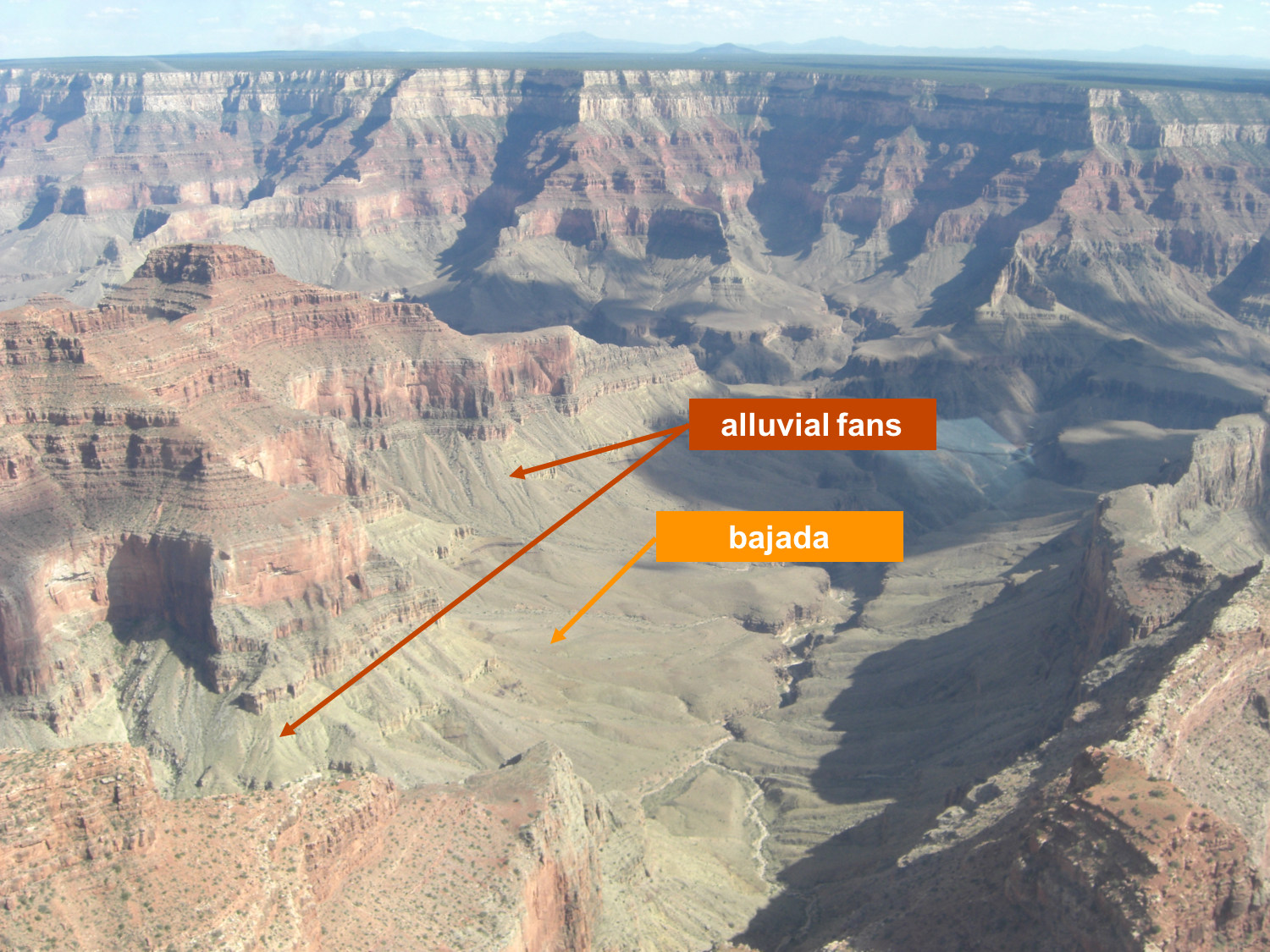
Common in more arid areas, alluvial fans consist of water-transported sediment that eroded en masse from breaks in a slope and formed a fan-shaped area of deposition in a valley. Areas susceptible to forming alluvial fans are generally denuded of vegetation or have very low vegetation density. The surface layer of soil is usually a very coarse material. A point at which multiple alluvial fans merge is called a bajada. Alluvial fans and bajadas, particularly in upper areas, may have diverse communities of shrubs and grasses, particularly in areas where rills form and channel water.
Section 4: Soil
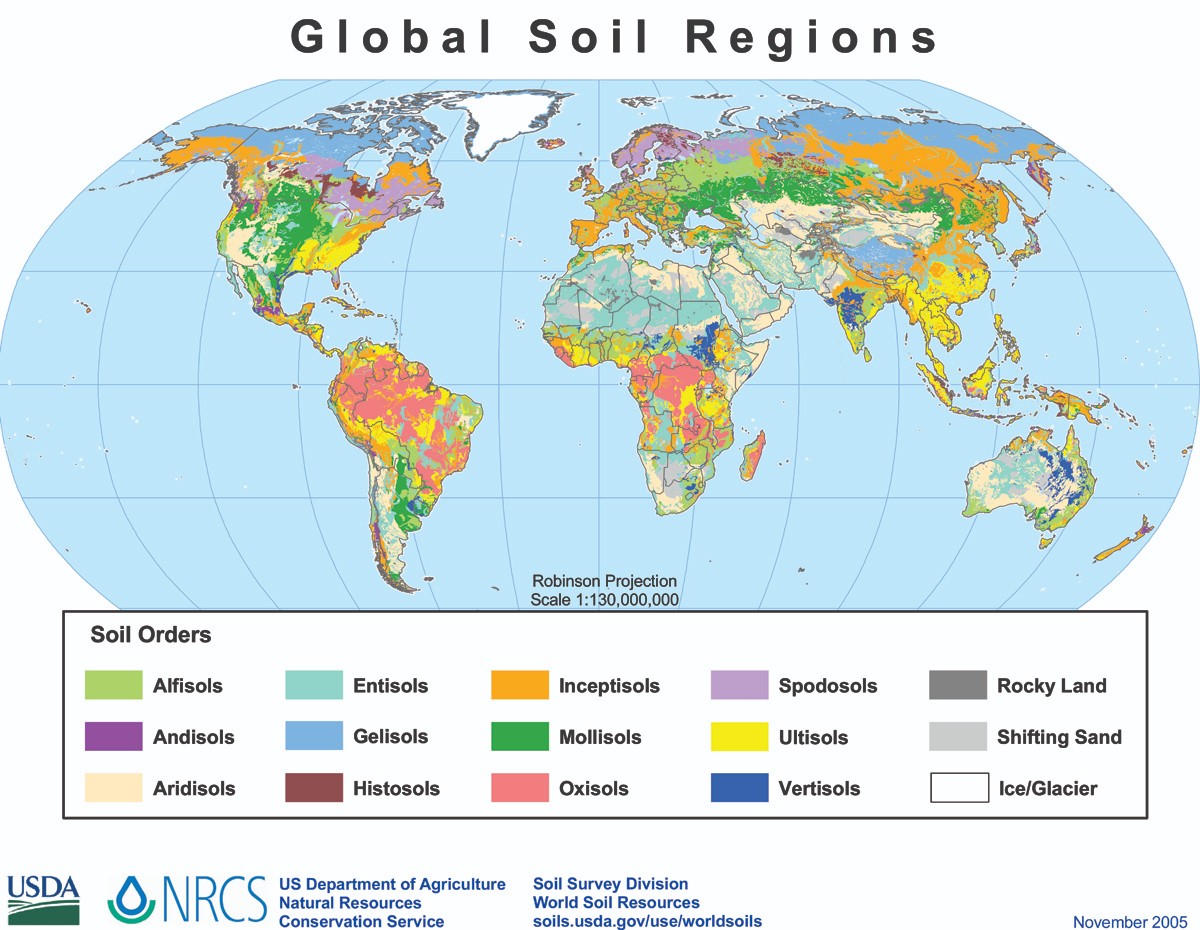
While precipitation is the primary determinant of vegetation production, soil is the primary determinant of the type of plant species present in a given area. For example, most desert soils are Aridisols or Entisols that are low in organic matter and high in mineral content and support plants adapted to drought, salinity, or high levels of calcium carbonate. The influence of soil is determined by the following:
- Parent material
- Soil organic matter (SOM)
- Texture and structure
- Salinity and pH
- Physical and biological crusts
Parent Material
Recall primary succession and the soil formation process. Parent material (sedimentary, igneous, and metamorphic rock) weathered over extended periods of geologic time; then, as plant communities developed and succession continued, soil horizons formed as organic matter mixed with parent material. In many systems with relatively shallow “B” and “C” horizons, bedrock (i.e., parent material) is still a substantial influence on soil characteristics.
Organic Matter (SOM) Parent Material
The fertility of a system is reflected in the amount of soil organic matter (SOM) it contains, a mixture of decomposing roots, microbial residue, plants, and animals that is rich in carbon and varying concentrations of nutrients. The amount of SOM influences the degree of nutrient cycling and carbon sequestration, soil structure and plant rooting, water infiltration and holding capacity, and available habitat for microbes.
SOM comprises light and heavy fraction components. Light fraction is recently dead material in the early stages of decomposition; it provides nutrients for plants and food for soil microbes. Heavy fraction has two types—physically protected and chemically stable—both of which contribute to soil structure and stability, water-holding capacity, and nutrient sequestration.
The amount and rate of SOM in a system depend on the amount of productivity, which in turn depends on rain and temperature. Hot deserts therefore generally have very little organic matter, while shrublands and woodland savannas have varying levels of organic matter, ranging from little in sandy saltbush desert to moderate in the sagebrush steppe and woodland areas of the Colorado plateau and California.
Texture and Structure
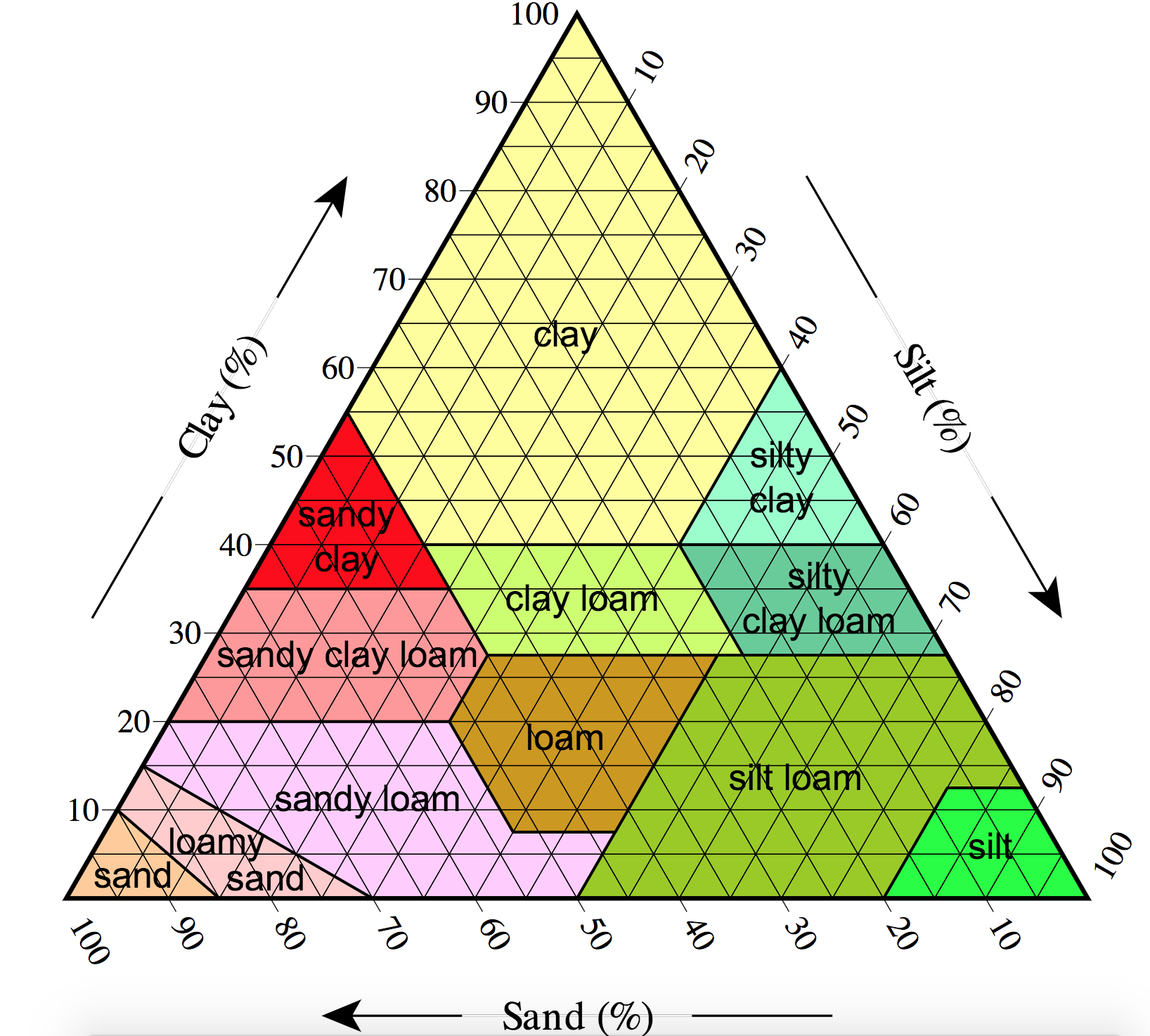
Soil texture is determined by the percentage of sand, silt, and clay in the soil composition. Sand particles are composed of silica and are generally large. Soil that is predominantly sand has large pore sizes and thus does not hold water or nutrients. Because silt particles are smaller than sand particles, silt has a much higher water-holding capacity (WHC) than sand. Silt particles are composed of minerals that weather quickly and release nutrients. Clay particles, the smallest of the three, have a great capacity to absorb and hold water in the soil profile, making much of that water unavailable to plants. Most soil combines two or all three types of soil particles and therefore has varying WHC and nutrient availability.
The term soil aggregate refers to the arrangement of soil particle units. Units have one of four shapes: spheroidal, platy, prism-like, and blocklike. Aggregate stability refers to the ability of an aggregate to resist degradation. Aggregate stability is determined by the soil texture and the amount of SOM present in the aggregate. The stability of aggregates may differ when wet or dry, and aggregates are susceptible to degradation by wind, water, and other physical disturbances, such as pressure from human activities. Aggregate stability is a key to erosion resistance, water infiltration, nutrient availability, and ease of root growth.
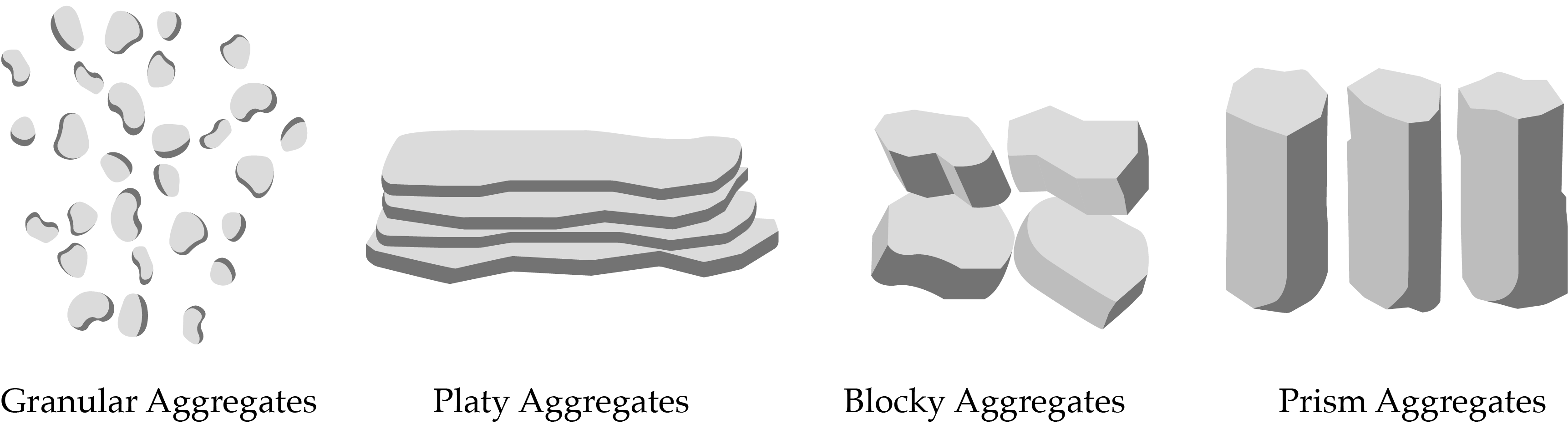
In systems such as sandy deserts where the small, spheroidal aggregates break apart easily, the risk of soil erosion is high. Systems with platy aggregates also have a high risk of edge erosion. Systems with prism-like or blocky aggregates, however, have reduced risk of soil erosion.
Soil Crusts
Arid and semiarid ecosystems often develop physical, biological, or chemical crusts. A physical crust is a thin, nonliving layer of mostly mineral content with low porosity. These crusts have low aggregate stability and are generally the result of a high degree of aridity and soils subject to erosion. Physical crusts limit water infiltration, thus reducing the water available to plants and impeding seedling emergence.
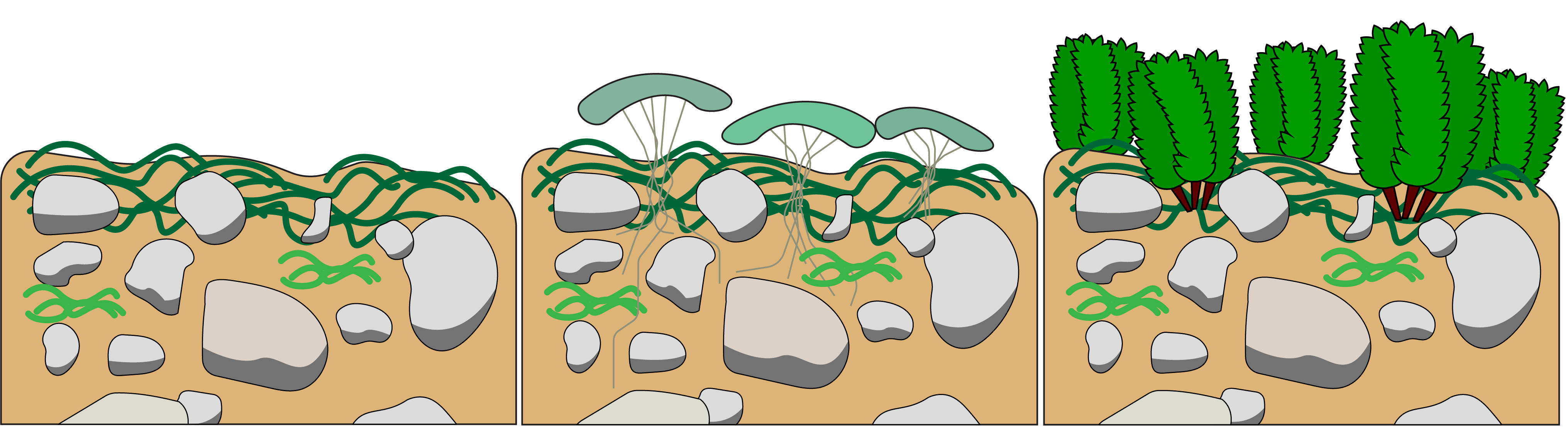
Biological crusts are living crusts comprised of interwoven communities of cyanobacteria, lichens, and moss. These crusts facilitate water infiltration, nutrient cycling, and soil-surface stability. Biological crusts generally form in arid ecosystems, or in systems spanning the border between arid and semiarid conditions, where soils are calcareous (possessing high concentrations of calcium carbonate) or gypsiferous (with high gypsum concentrations), as both these types of soils tend to limit plant growth.
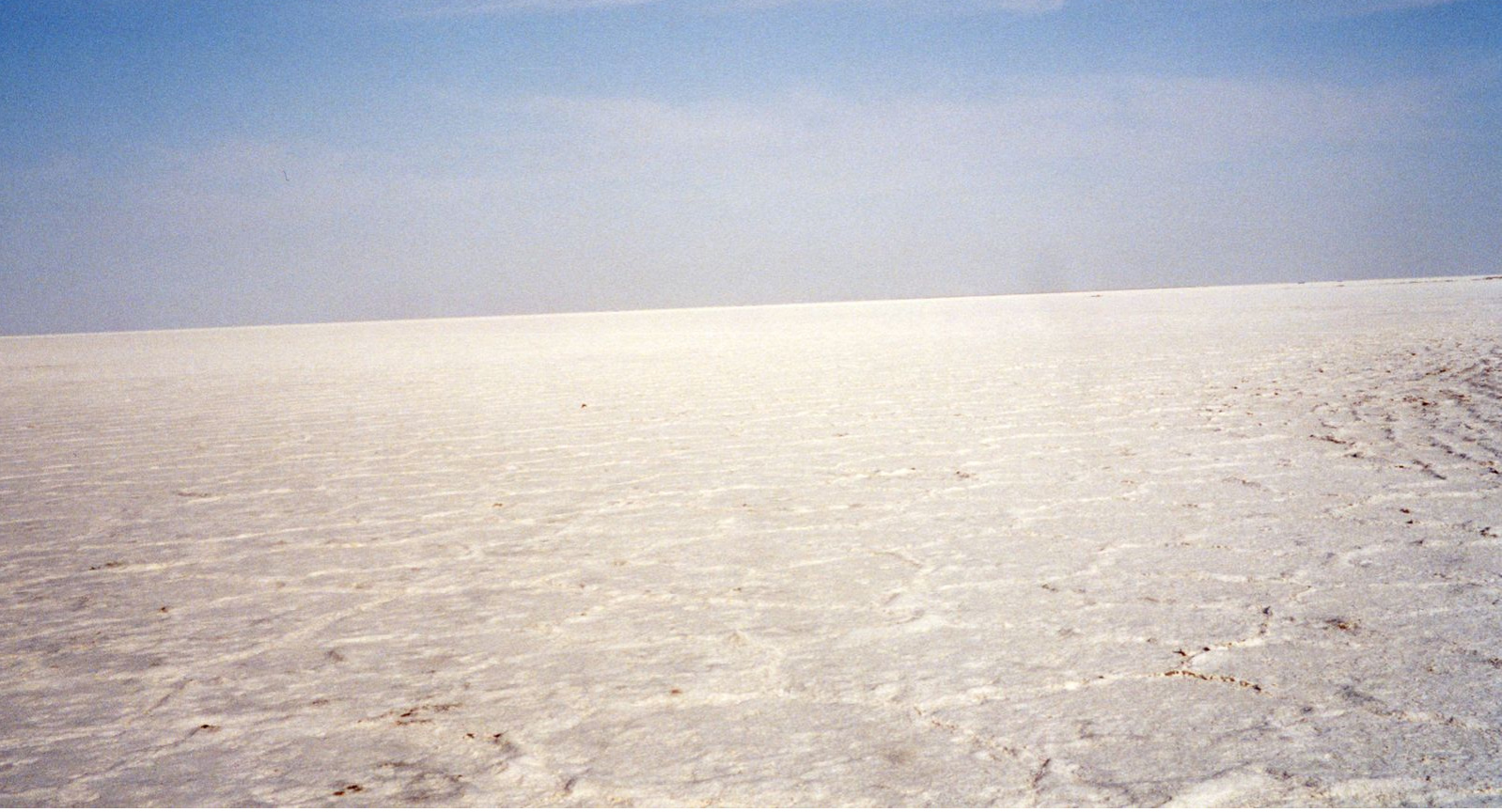
The composition of organisms that form the biological crust is influenced by climate, soil texture, and disturbance history.
Chemical crusts are thin layers of salt residue left after evaporation. This type of crust is found in the Great Salt Desert in the western United States. Conditions are very arid, and the soils are highly saline.
Conclusion
Biogeography is the study of the geographic distribution of life on Earth. It provides a framework for understanding why certain species occupy specific geographic ranges. The biogeographic framework encompasses climate (solar radiation, air and water circulation patterns, precipitation, temperature) and geology (soils, landforms, and topography). In the next few chapters, we will add an understanding of autecology and synecology to understand the biological and ecological structure and function of deserts, shrublands, and woodlands.