Unit 5: Exploring the Nature of Astronomical Phenomena in the Context of the Sun/Earth/Moon System
VI. Developing Additional Central Ideas Based on Evidence about the Sun, Earth, and Stars
This section focuses upon observing seasonal differences in patterns of stars that are visible at night, tracking details about the Sun’s apparent daily motion, and noticing seasonal differences in regional climates. Interpreting these observations will be helpful in developing an explanatory model for the Earth’s annual motion around the Sun as well as for explaining why the Earth has seasons,
A. Noticing seasonal patterns in the night sky
People who live where there are few lights at night typically are very familiar with patterns of stars that are visible in the night sky and with ways in which these change with the seasons. People who live in cities typically are unaware of such patterns and their changes because of the many artificial lights that brighten the night sky. Some star patterns may be visible, however, if you look up on a clear night. During this course, try to do this frequently!
Question 5.33 What seasonal patterns are evident in the constellations of stars visible at night?
- Discuss with your group members any patterns of stars that you have noticed.
- On a clear night go outside and look up! Enjoy what you can see and create your own patterns among the visible stars.
- If interested in what others have envisioned when viewing the stars, go to a website such as https://www.windows2universe.org/the_universe/Constellations/constnavi.html or
http://www.astronomy.com/observing/astro-for-kids/2008/03/learn-the-constellations where you can read about the circumpolar constellations, those which are visible all year long, and about constellations only visible during different seasons (spring, summer, fall, winter) in the northern and southern hemispheres. - To access an interactive map of the sky that can display what you might see on a clear night in your location, go to https://www.skyandtelescope.com/observing/interactive-sky-chart/. Click to open, enter your zipcode, year, month, day, and hour. To access names of bright stars, click the display option Star Names. To see traditional constellations, click on Constellation lines and later Constellation names.
Some constellations are visible in both the northern and southern hemispheres although the southern hemisphere constellations may look upside down from those shown below. Stars within a constellation look as if they are near by one another as seen from Earth; however, they likely are very distant from one another in space. In 1922, the International Astronomical Union defined 88 official constellations, (https://www.iau.org/public/themes/constellations/). Many of their names were derived from Greek and Roman mythology as shown in the star chart for the northern hemisphere in Fig. 5.50. For a similar star chart for the southern hemisphere see: https://maas.museum/app/uploads/sites/6/2016/03/starmapApril2016.pdf.

People of many cultures have seen a wide variety of patterns in the stars. The Ojibwe People of Canada and northern United States, for example, envisioned constellations based upon their own stories as shown in the Ojibwe Sky Star Map at https://web.stcloudstate.edu/aslee/OJIBWEMAP/home.html. This star map shows Ojibwe constellations superimposed on those described in Greek and Roman myths.
- Different constellations are high in the night sky during different seasons of the year. During spring in the northern hemisphere or autumn in the southern hemisphere, for example, look for stars that seem to form a backwards question mark as shown in Fig. 5.51. These stars were seen as outlining the head and mane of Leo, a constellation representing a vicious lion fought by Hercules according to Greek and Roman mythology. The brightest star, Regulus, has the Latin name for king. Another bright star, Denebola, has an Arabic name for “lion’s tail” (see: https://africancosmosdiary.wordpress.com/tag/constellations/). Many cultures interpreted the changing patterns of stars high in the sky in terms of seasonal patterns in climate. Seeing the constellation Mishi Bizhiw, a mountain lion , high in the sky, for example, warned Ojibwe travelers not to trust thin ice on lakes as they moved from winter camp (See information about Mishi Bizhiw at https://web.stcloudstate.edu/aslee/OJIBWEMAP/OjibweConstellationGuide.pdf).
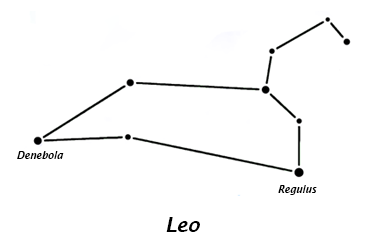
- During summer in the northern hemisphere or winter in the southern hemisphere, look for stars high in the sky that seem to form a half circle near stars that seem to outline a human figure as shown in Fig. 5.52. In Greek and Roman mythology, Corona Borealis was a northern crown and Hercules was a hero. In 2013, the biggest super cluster of galaxies in the universe, the Hercules-Corona Borealis Great Wall, was discovered in the direction of these two constellations (see https://www.space.com/33553-biggest-thing-universe.html). The Ojibwe community saw this half circular star pattern as representing Madoodiswan, a sweat lodge, with a person nearby, Noondeshin Bemaadizid, a bather exhausted from the experience but renewed in strength and spirit. (See: https://web.stcloudstate.edu/aslee/OJIBWEMAP/OjibweConstellationGuide.pdf).
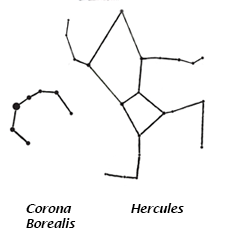
- During fall in the northern hemisphere or spring in the southern hemisphere, look for stars that seem to form a square near stars that seem to outline a flying bird as shown in Fig. 5.53. In Greek and Roman mythology, Pegasus, was a winged horse and Cygnus, a swan. Telescopes looking in this direction have detected planets orbiting stars outside our solar system (See: https://exoplanets.nasa.gov/resources/231/hd-209458b/). In Ojibwe mythology, Mooz was a moose, appreciated for providing food, clothing, and shelter. Ajiijaak was a crane, leading people to stay strong. (See: https://web.stcloudstate.edu/aslee/OJIBWEMAP/OjibweConstellationGuide.pdf).
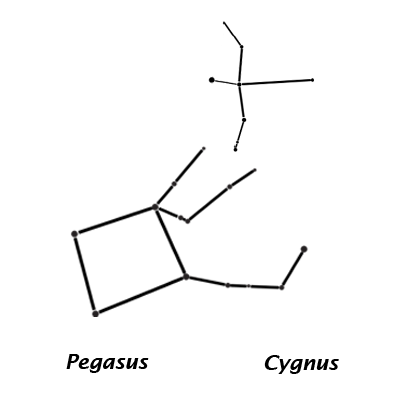
- During winter in the northern hemisphere or summer in the southern hemisphere, look for stars that seem to form a human figure with a belt as shown in Fig. 5.54. Orion was a great hunter in Greek mythology. Some stories envisioned Orion as followed by one of his dogs, Canis Minor, as he was raising a club and holding up a shield to fight Taurus, a charging bull (See: http://www.ianridpath.com/startales/orion.htm). Below the belt is M42, the Orion Nebula, where stars are being formed (https://www.nasa.gov/feature/goddard/2017/messier-42-the-orion-nebula). The Ojibwe community also envisioned a human-like figure, Biboonkeonini, the Wintermaker, with stars for shoulders and knees as well as three stars for a belt and long arms stretching to a bright star on either side (see: https://web.stcloudstate.edu/aslee/OJIBWEMAP/OjibweConstellationGuide.pdf).
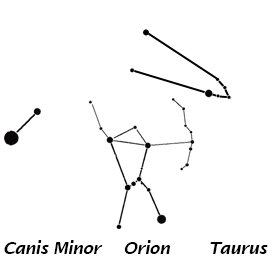
- Summarize the changes that occur in the constellations visible in the sky during a year. Complete entries in Table V.9. Then write a summary of what you have learned about seasonal differences in the stars visible at night.
TABLE V.9 Developing central ideas about seasonal differences in visible stars | |||
---|---|---|---|
Sketch of set up | Evidence | Central Ideas | Relevant Vocabulary |
Like the Sun and the Moon, the stars seem to be revolving around the Earth each night | constellation | ||
Some constellations of stars are seasonal, seeming to rise in the east and set in the west like the Sun and Moon but are only visible during part of the year | |||
Some constellations of stars are always visible in one hemisphere but not in the other. | northern hemisphere
southern hemisphere |
||
Some constellations are visible in both the northern and southern hemispheres depending upon where one is |
B. Noticing seasonal patterns in sunlight and shadows
In addition to seasonal differences in some of the stars visible overhead at night, there are large seasonal differences during the day in the lengths of shadows on the ground as well as in the Sun’s apparent motion across the sky.
Question 5.34 What seasonal patterns are evident in how the Sun seems to move across the sky?
1. Interpreting changes in the Sun’s maximum angular altitude α
Section III.B. Question 5.8 explored how the Sun seems to move across the sky by observing the shadows cast by a gnomon (person, post, paperclip, or nail) perpendicular to the ground as shown in Figs. 5.9 and 5.13 (repeated below).
- What do you wonder about such shadows?
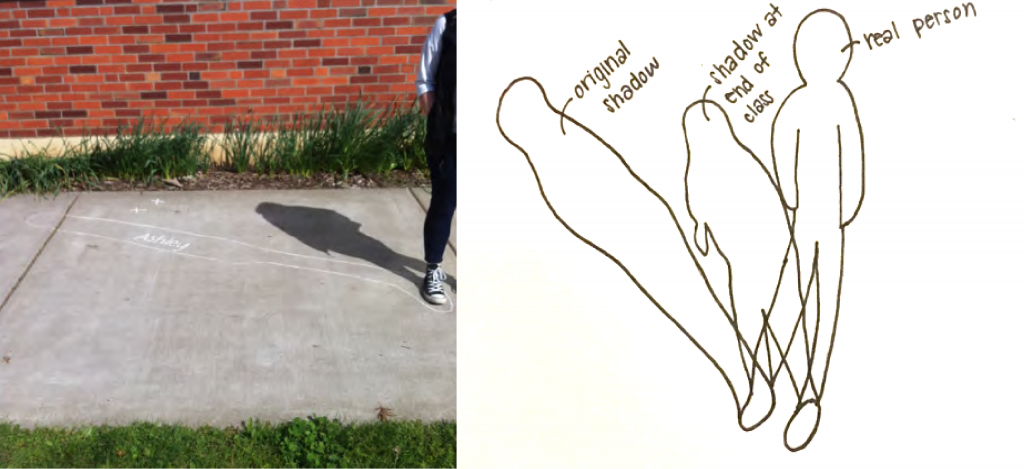
Some aspects to consider include:
- How do shadows change in size and orientation during morning, noon, and afternoon on a sunny day?
- In what directions do the shadows point?
- When during the day are shadows longest? When are shadows the shortest?
- What happens during the year? How do shadows change in size and orientation throughout the day during different times of year?
- How does the length of the shortest shadow during the day change with the seasons?
- What is the connection between how long a shadow is and how high the Sun seems to be in the sky?
Now consider how the Sun seems to be moving. DO NOT LOOK DIRECTLY AT THE SUN!
- What do you wonder about the Sun’s apparent daily journey across the sky?
Here are some aspects to consider:
- How does the Sun’s maximum angular altitude α (alpha) seem to be changing over a week? Over several weeks? Several months?
- Does the Sun’s apparent maximum angular altitude α seem to be getting higher? Lower?
- Is the Sun ever directly overhead?
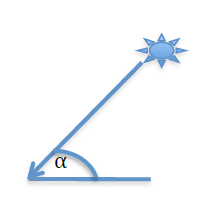
The angular altitude of the Sun, angle α (alpha), can be estimated by measuring the height of a gnomon H and the length of its shadow L, drawing a careful ray diagram and measuring the angle α with a protractor or calculating the tangent H/L, and identifying the angle for which that is the tangent as shown in Fig. 5.10 (repeated).
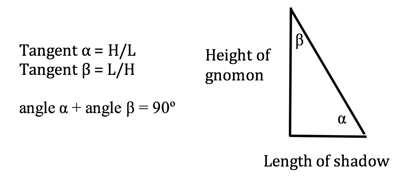
- Complete entries in Table V.10. Then write a summary of what you have learned about seasonal differences in the lengths of shortest shadows during the day.
TABLE V.10 Developing central ideas about seasonal differences in the lengths of the shortest shadows during a day | |||
---|---|---|---|
Sketch of set up | Evidence | Central Ideas | Relevant Vocabulary |
The shortest length of a shadow cast by a gnomon occurs when the Sun is at its apparent maximum angular altitude | gnomon
angular altitude |
||
The Sun’s apparent angular altitude α (alpha) is formed by the rays of the Sun and a gnomon’s shadow. Angle α can be estimated by measuring the height of the gnomon H, and length of the shadow L, drawing a careful ray diagram, and measuring α with a protractor or using a calculator or trig table to find the angle for which the tangent is equal to H/L. | protractor
tangent |
||
The angle β (beta) is formed by the rays of the Sun and a gnomon. This angle can be estimated by measuring β on a careful ray diagram with a protractor or estimated by finding the angle for which the tangent is (L/H). |
2. Interpreting data obtained from Internet resources
- What differences have you noticed in what the Sun seems to be doing during the different seasons of the year. During this course, for example:
- Where has the Sun seemed to rise? (north of east? directly east? south of east?)
- What has been the apparent daily maximum height of the Sun above the horizon? (high, medium, low?)
- Where has the Sun seemed to set? (north of west? directly west? south of west?)
- What is the duration of daylight compared to darkness? (longer time period? about the same time period? shorter time period?
- Use the Internet or other source of information to identify the current dates in your location for the:
- spring equinox (equal number of hours of sunlight and darkness)
- summer solstice (longest number of hours of sunlight and shortest number of hours of darkness
- autumn equinox (equal numbers of hours of sunlight and darkness)
- winter solstice (shortest number of hours of daylight and longest number of hours of darkness)
- To consider seasonal effects at your location, go to https://www.timeanddate.com/sun/ , enter a place or city in the search window, and explore predictions of what happens on the spring equinox, summer solstice, fall equinox, and winter solstice at your location:
- Where does the Sun seem to rise (north of east? east? south of east?) at this location on the spring equinox? summer solstice? autumn equinox? winter solstice?
- What is the maximum altitude of the Sun in the sky for this location on these dates?
- Where does the Sun seem to set (north of west? west? south of west?) at this location on these dates?
- What is the duration of daylight for this location on these dates?
- Create a table to report this information.
- Summarize these seasonal effects for your location for the Sun’s apparent:
- rising and setting times
- maximum height of the Sun’s arc across the sky
- duration above the horizon
3. Example of interpreting Internet data about changes in the Sun’s apparent daily motion
At https://www.timeanddate.com/sun/, enter Corvallis, Oregon, for example, in the search window for place or country, click on the Sunrise & Sunset button, change the month to March and current year, click on Go and click on a box in the row highlighted for the date of the equinox. A report appears as shown in Fig. 5.56. The green band indicates the start of daylight saving time at this location.
The report below predicts that on this spring equinox in Corvallis, the Sun would appear to:
- rise at 7:16 am, Pacific daylight saving time
- rise almost directly east (specifically 89° east of north)
- be highest in the sky at 1:20 pm time, Pacific daylight saving time
- be at a maximum altitude of 45° above the horizon
- set at 7:25 pm, Pacific daylight saving time
- set almost directly west (specifically 271° west of north)
and that the duration of daylight would be about 12 hours.
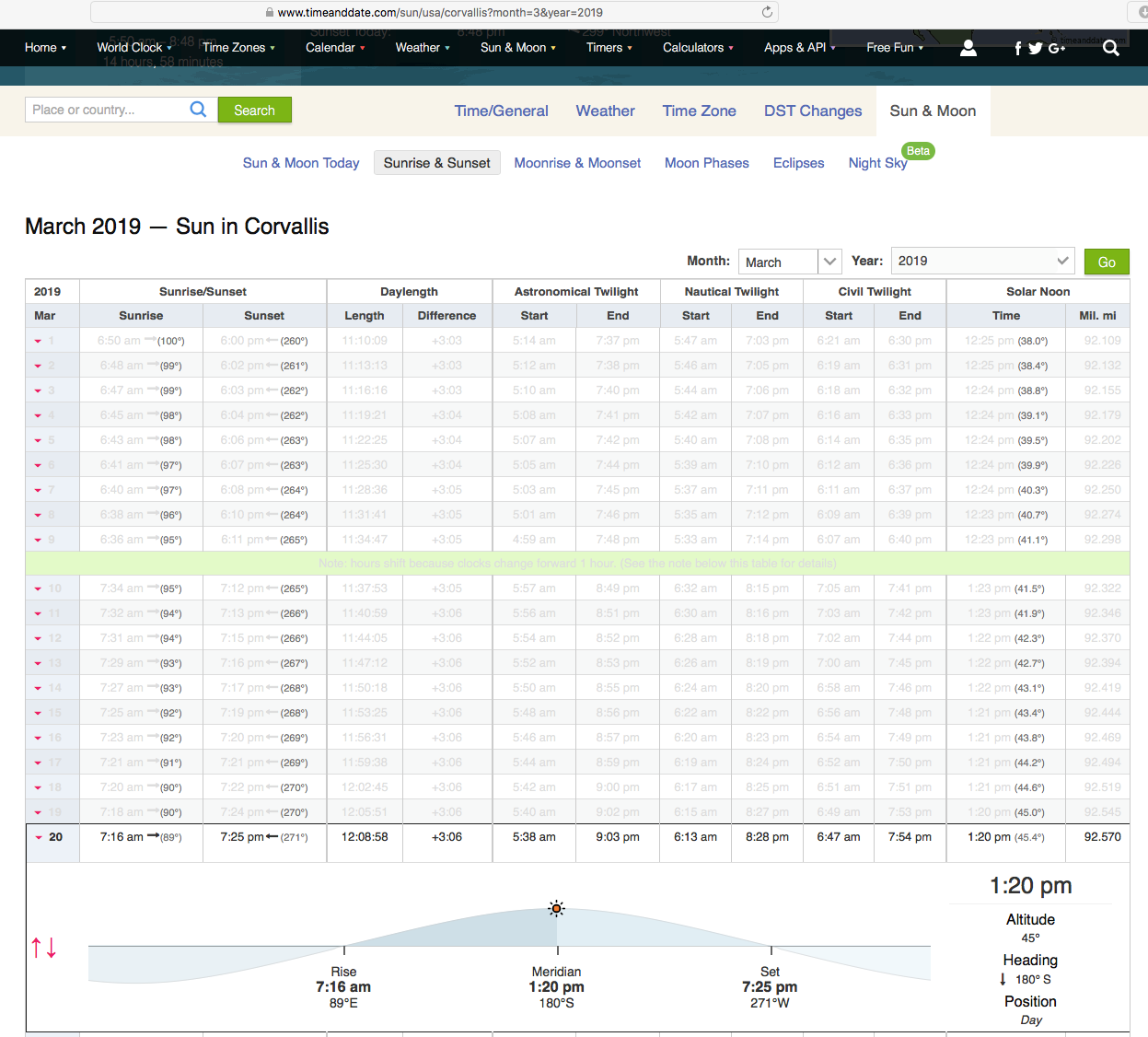
As shown in Fig. 5.57, predictions for these events on a June solstice in Corvallis, Oregon are for the Sun to appear to:
- rise at a much earlier time, at 5.28 am, Pacific daylight saving time
- rise north of east (55º NE)
- be at a much higher maximum altitude in the sky (69º) at about the same time (1.14 pm, Pacific daylight saving time)
- set much later, at 9:01 pm, Pacific daylight saving time
- set north of west (305º NW)
and that the duration of daylight would be much longer, about 15 ½ hours.

As shown in Fig. 5.58, predictions for these events on a September equinox in Corvallis, Oregon are similar to the March equinox. The Sun appears to:
- rise at about the same time, at 7:01 am Pacific daylight time,
- be almost directly east (89º E),
- be at about the same maximum altitude in the sky (45º) at about the same time (1.05 pm Pacific daylight time),
- set at about the same time , at 7:08 pm Pacific daylight time),
- set directly west (270º W)
and that the duration of daylight would be about 12 hours.

As shown in Fig. 5.59, predictions for these events on a December solstice in Corvallis, Oregon are for the Sun to appear to:
- rise at a much later time, at 7:46 am Pacific standard time, (equivalent to 8:46 am, Pacific daylight saving time)
- rise south of east (123º ESE),
- have a much lower maximum altitude in the sky (22º) at about the same time, 12:11 pm, Pacific standard time, (equivalent to 1:11 pm Pacific daylight saving time),
- set much earlier, 4:35 pm, (equivalent to 5:35 pm Pacific daylight saving time),
- set south of west (237º WSW),
and that the duration of daylight would be much shorter, about 8 3/4 hours.

The shaded portions of Figs. 5.56-5.59 demonstrate the large differences between the solstices in the duration of daylight and the maximum altitude of the Sun at this location. The information provided in this website is summarized in Table V.11.
Table V.11 Solar data for Corvallis, Oregon during equinoxes and solstices | |||||||
---|---|---|---|---|---|---|---|
Date in 2019 | Sun rise | Direction | Transit | Maximum angular altitude | Sun set | Direction | Duration of daylight |
March 20 spring equinox |
7:16 am
PDT |
89°
E of N |
1:20 pm PDT | 45° | 7:25 pm PDT | 271°
W of N |
12 hours |
June 21 summer solstice | 5:28 am PDT | 55°
N of E |
1:20 pm
PDT |
69° | 9:01 pm PDT | 305°
N of W |
15.5 hours |
Sept 23 autumn equinox | 7:01 am PDT | 89° E | 1:05 pm PDT | 45° | 7:08 pm
PDT |
270°
W |
12 hours |
Dec 21 Winter solstice |
7:46 am PDT | 123°
S or E |
12:11 pm PST
(like 1:11 pm PDT) |
22° | 4:35 pm PST (like 5:35 pm PDT | 237°
S of W |
8.75 hours |
The data provided in Figs. 5.56 – Fig. 5.59 and Table V.11 indicate that at our location, the Sun’s maximum altitude α (alpha) at the summer solstice is about 69º, but only about 22º at the winter solstice, and in between these, about 45º, at the spring and autumn equinoxes. What this means is that the shortest shadows during a day also change in length, being shortest at the summer solstice and longest at the winter solstice as shown in Fig. 5.60.
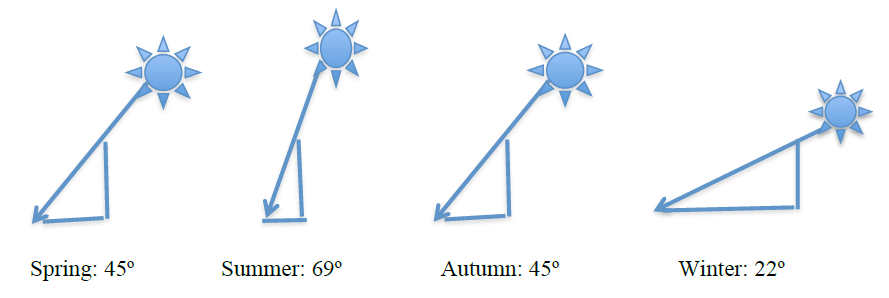
The data in Table V.11 also indicate that at our location:
- The Sun rises well North of East and sets well North of West at the summer solstice but rises well South of East and sets well South of West at the winter solstice, and in between these, rises in the East and sets in the West at the spring and autumn equinoxes.
- The Sun is above the horizon for the most hours (15.5) at the summer solstice and the fewest hours at the winter solstice (8.75), and in between these, above the horizon the same number of hours (12) as below the horizon at the spring and autumn equinoxes.
- During summer, the Sun’s maximum altitude is higher, the Sun’s rays shine down more directly through the atmosphere, and the duration of daylight is longer than during winter.
4. Cultural examples of noticing changes in the Sun’s maximum angular altitude α
Ancient peoples erected monuments oriented to the solstices such as at Maeshowe, built by Neolithic farmers about 5000 years ago on the Island of Orkney in Scotland. This burial cairn is a large mound made of stone, with a long low entrance passageway that is oriented so that sunlight shines through it into the inner chamber only during a few days before and after the winter solstice. ( http://www.orkneyjar.com/history/maeshowe/solstice.htm, http://www.orkneyjar.com/history/maeshowe/index.html, http://www.maeshowe.co.uk).
As shown in Fig. 5.61, ancient peoples also constructed large upright stone monuments oriented toward the Sun and Moon, such as the Calanais Standing Stones on the Island of Lewis, also in Scotland (https://stonesofwonder.com/callanis.htm).
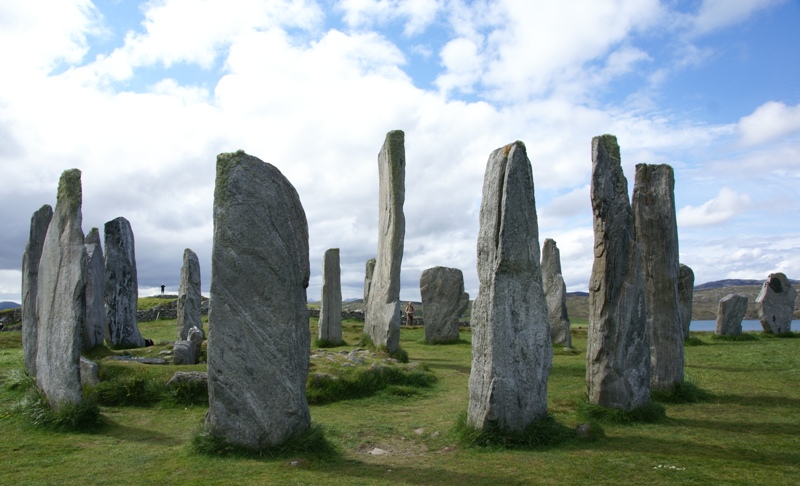
These sites predate Stonehenge in England, which also has large upright stones positioned with astronomical significance (https://www.english-heritage.org.uk/visit/places/stonehenge/history-and-stories/history/significance/). New instruments and techniques have led to new information about this area (https://www.smithsonianmag.com/history/what-lies-beneath-Stonehenge-180952437/). Recent droughts have led to new discoveries in Ireland of the remains of ancient henges, circular structures that have become visible in fields photographed from above by drones (https://www.smithsonianmag.com/smart-news/drought-reveals-giant-4500-year-old-irish-henge-180969650/).
Several ancient henges in North America have been excavated in part of the Cahokia Mounds State Historic Site located in Collinsville, Illinois. Built about 900-1100 A,D, these henges were made with red cedar posts about 15-20 inches in diameter and about 20 feet high. Some aligned with the rising Sun during solstices and equinoxes. See the description of WoodHenge at https://cahokiamounds.org/explore/#tab-id-r.
Modern people have chosen to celebrate astronomical solar equinoxes and solstices in similar ways. In a project led by astronomer Judith Young, the University of Massachusetts at Amherst has constructed a modern standing stone circle (See: http://www.umass.edu/sunwheel/pages/whatis.html) and http://www.umass.edu/sunwheel/images/sunwheel/paper.html
The Southern Colorado Astronomical Society has created a standing stone circle near Spanish Peaks in a project led by Russ Erganbright, a retired engineer. They have carefully sited each stone to illustrate where the Sun will rise and set during equinoxes and solstices. (See https://www.skyandtelescope.com/sky-and-telescope-magazine/beyond-the-printed-page/beyond-the-printed-page-a-modern-standing-stone-calendar/)
As shown in Fig. 5.62, Keppel Henge is a standing stone circle in a garden in Ontario, Canada that was developed by Steve Irvine and Bill Loney.
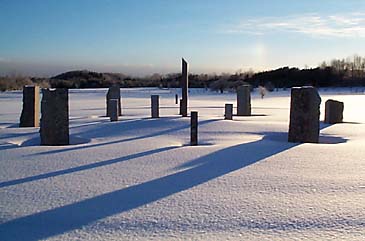
Keppel Henge includes a North Star Stone with a notch through which a nearby Pointer Stone points at Polaris, the North Star. As shown in Fig. 5.63, the stars appear to move around Polaris during this photograph made with a one hour exposure.
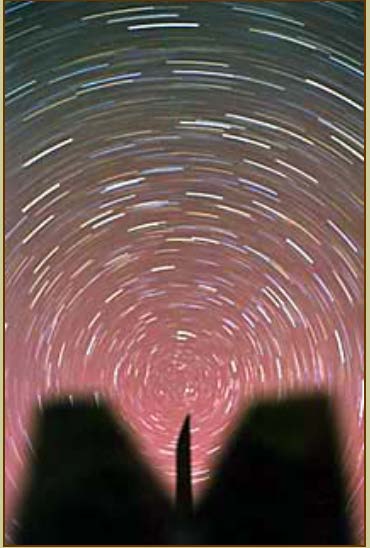
As shown in Fig. 5.64, Steve Irvine used a pinhole camera to photograph the apparent path of the Sun over a six-month period at the Keppel Henge site. This photograph shows the changing height of the arc that the Sun made as it appeared to move across the sky. The pinhole camera was a coffee can with a pinhole on one side, attached to the trunk of a tree.
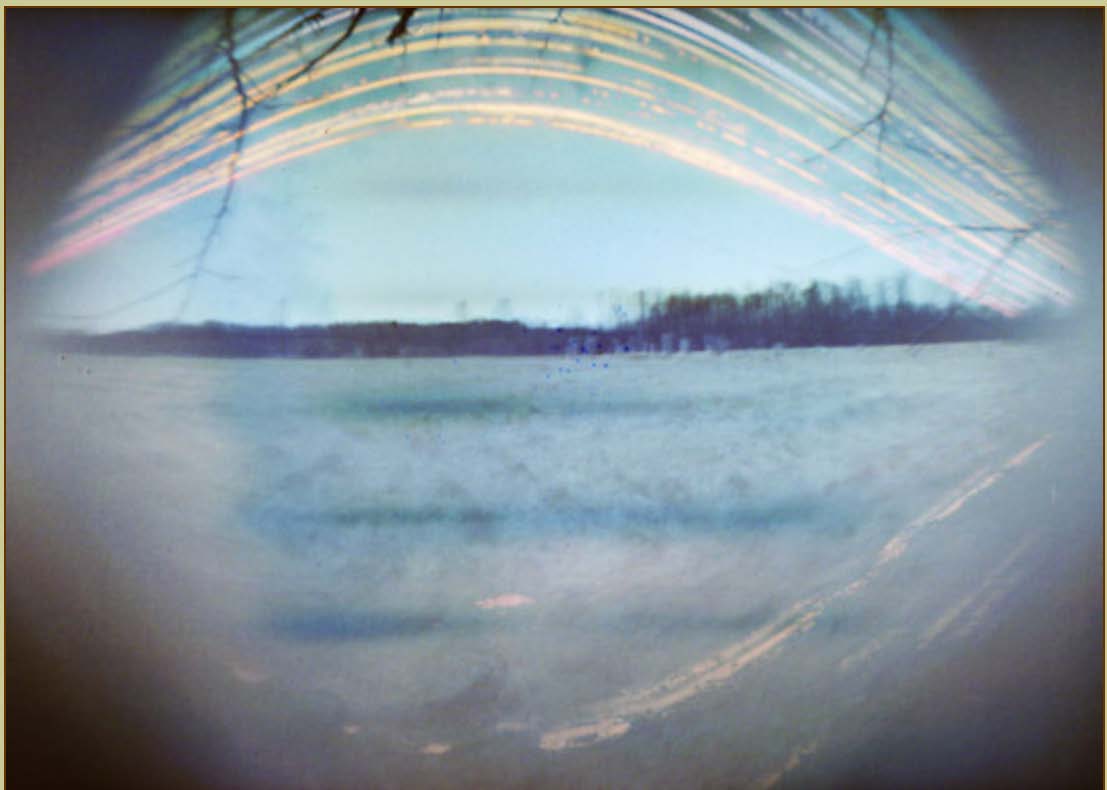
For additional images of the Sun, Moon, and stars from this location, see http://www.steveirvine.com/astro/index.html
C. Interpreting connections between seasonal differences in the Sun’s apparent angular altitude and regional climates
Question 5.35 What is the connection between seasonal differences in the Sun’s apparent angular altitude and regional climates?
- To consider monthly differences in climate in the US, click on your state and nearest town or city in https://www.usclimatedata.com/climate/united-states/us
- Describe seasonal differences in:
- average monthly temperature
- average monthly precipitation
As shown in Fig. 5.65, for example, a graph represents the average monthly high and low temperatures as well as monthly average rainfall for Corvallis, Oregon.
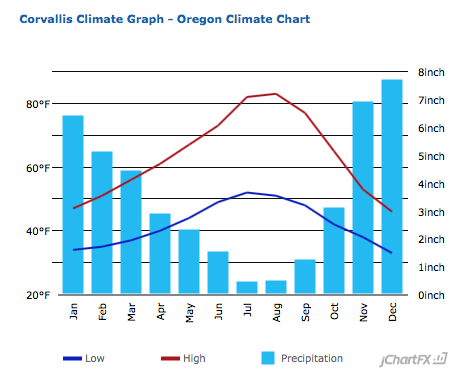
In summary, during summer in our location the Sun rises north of east and sets north of west during the spring and summer; rises directly east and sets directly west, during the equinoxes; and rises south of east and sets south of west, during autumn and winter. The Sun appears to rise earlier in the morning, to travel in a higher arc across the sky, and to set later in the evening in the summer than in the winter. The Sun’s maximum angular altitude is about 69º on the summer solstice, 45º on the equinoxes, and 22º on the winter solstice.
During summer the Sun is above the horizon for more hours and seems to travel in a higher arc across the sky so that its rays shine more directly down through the atmosphere. Therefore, more energy from the Sun reaches the surface for a longer time period during the summer compared to the amount of energy from the Sun reaching the surface during winter. Our summers are warm and dry; our winters cool and wet. This climate is typical for our region, the Pacific Northwest in the US.
For some world wide climate information, go to https://www.nnvl.noaa.gov/view/globaldata.html .
- Summarize the seasonal changes that occur in your location for:
- when and where the Sun appears to rise
- how high the Sun appears to go in its arc across the sky
- when and where the Sun appears to set
- how long the Sun is above the horizon
- the average monthly temperature
- the average monthly precipitation
Complete entries in Table V.12. Then write a summary of what you have learned about connections between seasonal differences in the details of the Sun’s apparent daily motion and in the typical temperature and precipitation experienced in your region on the Earth.
TABLE V.12 Developing central ideas about seasonal differences in the details of the Sun’s apparent daily motion and in regional climates | |||
---|---|---|---|
Sketch of set up | Evidence | Central Ideas | Relevant Vocabulary |
The Sun seems to rise north of east (spring, summer), directly east (spring and autumn equinoxes), and south of east (autumn and winter) in the northern hemisphere | |||
The Sun’s apparent highest maximum angular altitude (angle α) in the sky occurs at the summer solstice; the Sun’s lowest maximum angular altitude α occurs during the winter solstice. | |||
The Sun seems to set north of west (spring, summer), directly west (spring and autumn equinoxes), and south of west (autumn and winter) in the northern hemisphere | |||
During spring and summer, the Sun is visible above the horizon for a longer duration (more than 12 hours) than not visible below the horizon; On the spring and autumn equinoxes, the Sun is visible above the horizon for the same duration, 12 hours, as not visible below the horizon; During autumn and winter, the sun is visible above the horizon for a shorter duration (less than 12 hours) than not visible below the horizon. | |||
During summer, the Sun’s maximum altitude is higher, the Sun’s rays shine down more directly through the atmosphere, and the duration of daylight is longer than during winter. |