A New Nature
9 Earth Is Heaven
What Is Nature? Should We Manipulate and Manage It in Order to Save It?
Preserving the old ways from being abused
Protecting the new ways, for me and for you
What more can we do?
—The Kinks, The Kinks Are the Village Green Preservation Society
As geographic features go, the North Pole is more a cartographic concept than a definitive place. On a map it’s a point, but up close it’s an almost featureless jumble of constantly moving, forming, melting, and floating ice. It is such an amorphous destination that we still aren’t certain who among the host of explorers that raced to get to the pole first actually got there.1 Our collective imagination of the North Pole is probably more solid than the physical location. It symbolizes something removed from us. Cold. Extreme. Outside. Wild. But even that is beginning to get a bit fuzzy. We can now hop on a plane and take a summer trip to the North Pole with little more effort than it takes to book a European vacation.”2 Human-induced climate change along with the other forces of the Anthropocene are dramatically reshaping the arctic ecosystem (see Chaps. 5 and 6). The wildness of the North Pole is no longer strictly apart from us.
I grew up a long way from the North Pole, near a different wild place: a thin sliver of eastern deciduous forest in suburban Maryland. It had been slated to become a freeway, but over the years as budgets dwindled and priorities shifted, the freeway plan languished. The land sat there largely unnoticed and forgotten as the region around it grew increasingly urbanized. After school, before my parents came home from work, I would slip past the no-trespassing sign to spend hours exploring the forest. I imagined myself an explorer naturalist in the mold of Alfred Russel Wallace. I identified trees, documented aquatic life, collected curious rocks, unearthed strange relics, and a few times even convinced myself that I had become hopelessly and tragically lost. I never once saw another person in the forest, even though I was never much more than 100 meters from suburbia.
Years later, the freeway plan was permanently shelved, and the land was conserved as a state park named in honor of the arctic explorer Matthew Henson. Henson was a native son of Maryland, who may (or may not) have been the first person to reach the North Pole (Fig. 9.1). Even accounting for the cynicism that comes with age, I find it difficult to see much wildness in Mathew Henson State Park now. The new park boasts a paved hiking trail, informative signs, boardwalks, and emergency phones. Still, it is a place where we seek out nature. A place where we limit our actions to observing and appreciating the natural world.
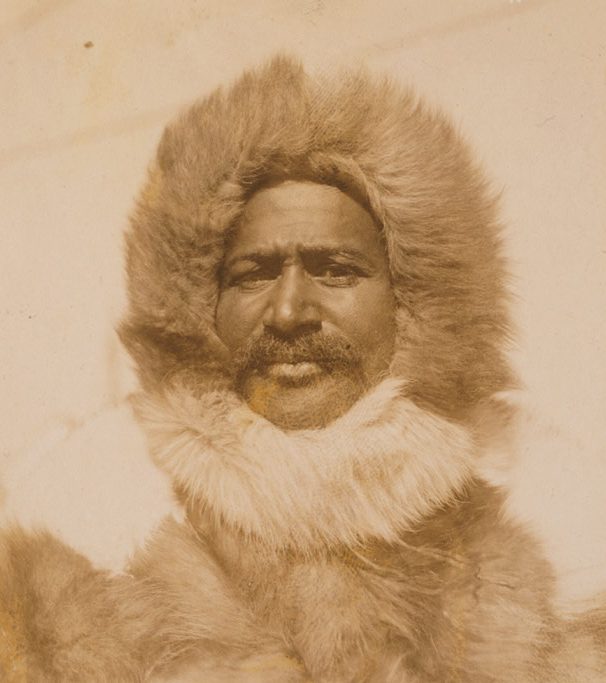
Whether by design or accident, much of the world is still wild in the sense that it is at least partially independent of our direct control. We increasingly value these natural places. As the previous chapters in this book imply, however, defining nature is getting increasingly difficult. Figuring out how best to preserve that naturalness is even more difficult. In the middle of the twentieth century, the discipline of conservation biology developed to help provide some answers and guidance. As the term advertises, conservation biology has focused on finding ways to conserve existing biodiversity from our diminishing actions. As such, it tended to take as models places and times when our influence on ecosystems was minimal. The rapid and ubiquitous changes occurring during the Anthropocene have forced conservation biologists to rethink their perspective, however. Perhaps the goal cannot be to preserve systems such as coral reefs or alpine forests in their current forms. Instead, perhaps we should ask what we want those systems and the rest of biodiversity to look like in the future. These concepts are at once subtle and profound. Certainly, in many cases, our vision for future biodiversity will include strong connections to the past and systems where we are only one equal part of a complex biological tapestry. But humans have become much more than an equal part of the tapestry. We have become its weaver.
In this chapter, I briefly explore some of the existential angst about what nature is and our relationship to it. I then outline two important components of conservation biology: restoration ecology and invasive species management. These subfields illustrate our changing perspectives as well as our still conflicted ideas about how we should conserve biodiversity. In Chapter 10, I explore some other approaches and strategies that push the boundaries of conservation biology even further as we struggle with stewarding nature through this human age.
9.1 What Is Nature?
Section 9.1: What Is Nature?
Our ideas of what nature is and our conceptions of our place in the universe are central aspects of human culture and society. They have been subjects of art, literature, and philosophy ever since we started to depict the world and ask questions about existence. How we framed and tried to answer questions about the world also strongly shaped the historical development of science. Those are some big, important, and complex topics. For the sake of space, not to mention my woeful lack of expertise, I’m not going to cover any of that here. See the additional resources for a tiny fraction of that broader exploration.
Instead, I’ll take a far briefer and more practical approach. We all have our own idea of what nature is—or perhaps more definitively what it isn’t. That includes ecologists, planners, and resource managers whose lines of study and approaches to management have been shaped in various ways by different perceptions of nature. For example, when I was in graduate school, a friend of mine wanted to study the ecology of orchids colonizing citrus orchards on a small Caribbean island. He was interested in the diversity of the colonizing orchid species, what their ecological niches were, and how they interacted with each other and the trees. My friend’s advisor thought the plan was insane. What could my friend possibly learn about the fundamental ecology of niches and competition by studying orchids in such an obviously human-manipulated and artificial system? The advisor thought the study might be appropriate if my friend wanted to become a lemon agronomist, but it was hardly suitable for a budding community ecologist.
We usually don’t think about what our definition of nature is and how it shapes our decisions. But the astonishingly rapid changes happening to the planet are forcing us to think critically about our assumptions and biases. In this section, I outline some of the main ways we currently define nature in (mostly) biological terms. That is perhaps an odd way to approach the definition. Nature is much more a cultural, religious, and philosophical concept than it is a biological one. By necessity, I will touch on those other aspects, but I won’t provide a comprehensive overview of them. These definitions (or broad concepts) are not mutually exclusive, and they usually get combined in various ways.
Everything
It is likely that our first conceptualization of the natural world was simply the world around us. Our earliest surviving works of art depict the world we lived in: animals, plants, the sun, moon, stars, and ourselves. In a cave on the Indonesian island of Sulawesi, a painting that is at least 45,500 years old depicts realistic images of Sulawesi warty pigs (Sus celebensis). The artwork includes a couple of stenciled human handprints, perhaps an early form of saying, “I was there. I saw that” (Fig. 9.2). Similarly old artworks in other nearby caves depict other local animals such as dwarf buffaloes (Bubalus sp.). There are also a few tiny figures that appear to be part human, part animal hunters. The art may not exactly be a journalistic record of events, but rather one of our first spiritual stories.3
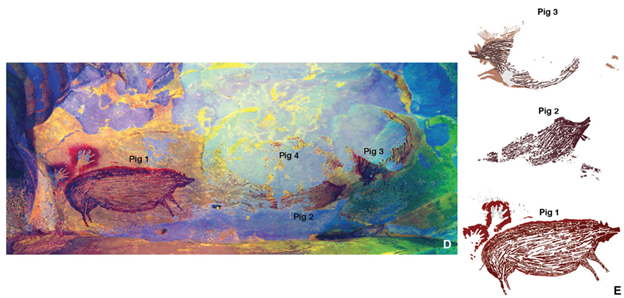
The paintings illustrate two concepts that have been central parts of our culture and religion for tens (perhaps hundreds) of thousands of years: (1) that nature is the world (including us) and (2) that nature is imbued with spiritual power and meaning. Many human cultures and spiritual beliefs involve aspects of animism. One common theme in animist beliefs is the idea that all aspects of the world—animals, plants, us, rocks, the wind—have unique spiritual essences. Another animist idea is that all the different physical and spiritual aspects of the world are connected in a complex web of interaction.4 Animism is still a central aspect of many Indigenous societies and traditional cultures. For example, the Waorani people of the western Amazon view the forest where they live as a spiritual place as much as a physical one. In Waorani culture, there is no distinction between the physical and spiritual aspects of the world; the forest is a manifestation of spirts that form intricate relationships with each other and with the Waorani.5 Aspects of animism have also been incorporated and adapted into globalized cultures. For example, Pope Francis uses them in Laudato si’, his encyclical on the environment. In it he argues, “The entire material universe speaks of God’s love, his boundless affection for us. Soil, water, mountains: everything is, as it were, a caress of God,” and “Nature cannot be regarded as something separate from ourselves or as a mere setting in which we live. We are part of nature, included in it and thus in constant interaction with it.”6
Over the past few hundred years, these holistic and spiritually focused views of nature have been joined by more mechanistic and human-centric views. A salient aspect of these humanist worldviews is that nature is a collection of phenomena that we can understand by making empirical observations and designing experiments. This concept tends to frame us in a special role: an intelligent, rational observer. That in turn tends to implicitly separate us from the natural world we are describing. Humanist concepts have become dominant aspects of human culture, and they have influenced the ecological, social, and economic changes taking place during the Anthropocene. The details of that are topics for another book—or a few hundred (see the additional resources). One aspect of that complex influence is that as humanist views of nature came to be an increasingly dominant part of global culture, they increasingly overwhelmed and marginalized ideas about nature from Indigenous and traditional cultures. In many cases, this was part of explicit programs to subjugate people, disposes them of land and resources, and strip them of their cultural identity.7 It also has involved more subtle biases, roadblocks, and cultural miscommunication that have often excluded local viewpoints and perspectives from research and resource management decisions.8
Absence of People
The absence of people is one way to define nature. After all, getting away from people is one of the main reasons many of us seek nature out.9 Despite the nearly 8 billion of us, we can still do that. There are large chunks of the planet where you are unlikely to see another person. Most of the world ocean is uninhabited. While uninhabited bits of land are much rarer, there are still many sparsely populated swaths—albeit mostly in extreme places such as the coldest parts of the cryosphere, the hottest deserts, and the wettest forests. Uninhabited or sparsely populated places have been where we traditionally focused ecological research and where we targeted conservation efforts.
Focusing on the absence of people has some complications, however. One is that the first step in nature conservation has often involved kicking out any of the few people who happen to be living there. This can sometimes align conservation with racism, inequity, and injustice. About half of the officially protected areas set up around the world since 1900 were occupied or regularly used by Indigenous peoples who were evicted when the reserves were created.10 The expulsions and exclusions are often based more on creating an unpeopled ideal than on well-documented ecological reasons. For example, in the 1930s, Uganda created three large forest reserves in the biodiverse southwest of the country. The Batwa people who lived in the new reserves were allowed to stay and continue their largely traditional lifestyle. But over the years, illegal activities such as wildlife poaching began to have severe negative effects, particularly for critically endangered mountain gorillas (Gorilla beringei beringei). Although the poaching and other illegal activities were largely driven by outsiders, the Batwa were wrongly accused of participating. Responding to pressure from conservation groups, Uganda formally designated the reserves as national parks in 1991 and forcibly evicted the Batwa.11 The case of the Batwa illustrates how the process of establishing a people-free reserve can be fundamentally unfair: a few privileged folks uprooting the lives of less powerful people who have little or no say in the matter. To make matters worse, the disenfranchised people often have the most vested interest in preserving the park’s ecology as well as the practical knowledge to do it.
Another challenge to the idea of nature without people is that an increasing number of us have the ability to visit remote and sparsely populated places. A significant segment of the tourism industry is focused, ironically, on allowing people to get away from people. Not surprisingly, the ability for more of us to travel to see natural areas and wildlife is causing some of the problems we implicitly are trying to escape: overcrowding, ecological damage, the exploitation of organisms. Examples from just before the COVID-19 pandemic locked down global travel include deadly traffic jams on the way to the summit of Mount Everest,12 the long-term closure of Maya Bay in Thailand to tourists because of the severe ecological damage they caused,13 and the trampling of wildflowers by selfie-seeking tourists during the 2019 California super bloom.14 Still, tourism can be an important way to support conservation efforts. It is particularly effective at channeling money more directly to the local stewards of conservation areas. Ecotourism emerged in the 1980s as a way to explicitly align tourism with the goals of conservation. Techniques to achieve that aim include rules and practices designed to limit the ecological impact of the visitors, employing local people as stewards and guides, using a portion of tourism revenue for conservation and outreach programs, and investing local communities with the power to make decisions on how to manage the tourism.15
Absence of Human Impacts
Another definition of nature is a pristine place unaffected by damaging human activities, not necessarily the absence of people altogether. This view of nature has strongly influenced our approach to management. The central part of creating any natural protected area is regulating human activities and narrowly prescribing the ways in which we can influence ecosystems. As the examples throughout the rest of this book illustrate, doing that is getting increasingly difficult, and in the most absolute sense it is already impossible. While many human impacts are generated locally, much of them are the result of truly global phenomena stemming from our collective actions. Climate change is the prime example, but other examples include the human-assisted spread of species around the world.16 and the global spread of a range of pollutants including plastic,17 aerosol particulates,18 and mercury.19 It is getting increasingly difficult to preserve nature simply by restricting human activities at the local scale of a reserve.
Another complication is that deciding which human activities are compatible with nature and which are not is subjective. Like many subjective things, that decision is shaped by society and culture. When Europeans colonized North America, they saw a vast, untouched wilderness. This is how William Bradford (governor of the Plymouth Colony) described his new home: “the whole country, full of woods and thickets, represented a wild and savage hue.”20 That wildness extended to the people who were already living there. Europeans often treated Indigenous people as parts of the natural world that either needed to be tamed and civilized or that represented an ideal of a noble savage uncorrupted by civilization. Those racial and cultural biases played no small role in how long it took scientists to appreciate the strong influence that Indigenous Americans had in shaping the ecology of the continent. Many parts of the landscape that Europeans first encountered were probably not untouched wilderness, but instead were actively managed using techniques such as prescribed burns, the draining of wetlands, and agroforestry.21
Differing views of what people see as acceptable activities in natural areas constantly complicate ecological management and conservation. Conflicts commonly arise over how and even if invasive species should be controlled, whether roads and other tourist infrastructure should be built, and whether to harvest native plants and animals. How these debates get resolved vary across cultures, local communities, and over time. A good example comes from Yosemite National Park. Up until it fell in 1969, the Wawona Tunnel Tree was actively promoted by the National Park Service as a must-see attraction (Fig. 9.3). But by 1969, when the tree fell, opinion on what was an appropriate activity in a national park was changing. The Park Service never considered carving out a replacement tree. There was, however, a debate about what to do with the downed tree. Suggestions included selling the tree for timber or turning it into various commemorative objects. The Park Service decided to leave the downed tree alone to provide habitat and to let the natural processes of decomposition and succession develop unhindered.
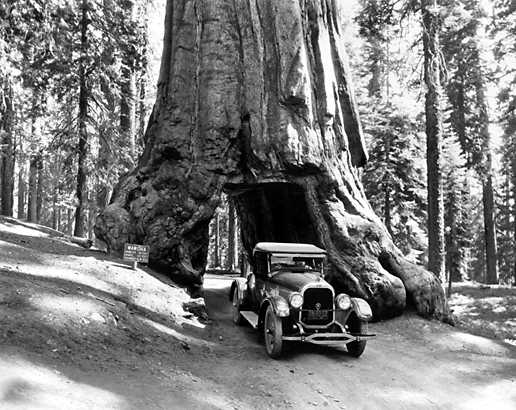
These types of conflicts continue to evolve. Today, many conflicts stem from the fact that more of us live adjacent to wildland areas along the wildland-urban interface. For example, homeowners often demand fire suppression in adjacent wildlands while opposing the use of prescribed burns as a management practice because of the air pollution it creates and because of fear the burns will get out of control.22
Species
One definition of nature is simply any living thing other than us. This definition includes the many species that live in close association with us, such as species that are parts of domesticated ecosystems, species that are products of domestication themselves, and species living in human captivity (e.g., zoos). Despite that, in some ways this is one of the least human-centric definitions of nature, and it shares some similarities with the everything concept. Nature always retains a part of its naturalness independent of how much we may shape or modify it. At the same time, we form intense connections with organisms. The biologist E. O. Wilson argued that we have an innate attraction to living things, a biophilia.23 For many people, a quiet spot in a city park, the vegetable garden they tend as a hobby, and the companionship provided by their pet all provide important connections to the natural world.
This view of nature is reflected in often seemingly contradictory approaches to how we interact with the natural world. On one hand, we lovingly tend and primp the living parts of our domesticated urban landscapes such as parks (Chap. 8). At the same time, we question our ethical right to exploit or manage species. These two sentiments often create conflicts. For example, our affection for domestic cats and dogs has created free-roaming populations that have a large impact on native biodiversity in many regions. But our affection for them also prevents us from effectively managing their populations. Attempts to control feral cat populations are often met with stiff human opposition.24 More subtly, the species view of nature also underlies approaches to ecological management that focus more on preserving certain functions of ecosystems and less on value judgments about the species making up the ecosystems. In these views, all species have innate value, and it is less important that they are native or non-native, rare or commonplace. An urban forest filled with weedy commonplace species could have just as much value as a remote forest filled with rare endemic species.
Self-Regulating
Perhaps the most ecologically framed definition of nature is an ecosystem whose characteristics are maintained through internal, nonhuman feedbacks and processes. One problem with that definition is that ecologists have struggled with what they mean by it. The opposite condition is actually easier to describe. Some ecosystems are maintained almost entirely by human actions and manipulations; the characteristics of these ecosystems change radically if human actions stop. Take for example an irrigated alfalfa field in southern Arizona. If we stop the inputs of water, fertilizer, and pesticides, the alfalfa field would quickly start to change—perhaps back into something resembling the Sonoran Desert it once was. The Sonoran Desert, in contrast, exists without—indeed in spite of—our manipulations.
But defining natural ecosystems such as the Sonoran Desert based on their autonomous stability is fuzzy. Part of the fuzziness relates to a question that ecologists have long struggled with: To what degree do ecosystems form a collective whole? In the early twentieth century, Frederic Clements was the main proponent of a model that described ecosystems as a kind of superorganism.25 Think about yourself. You are made up of millions of different individual cells. Yet they interact to form a unique collective entity: you. You have characteristics that clearly distinguish you from those of your component cells and from other individuals. Those traits are more or less stable—excepting a few poor fashion choices, bad haircuts, and extra pounds over the years. Most importantly, natural selection acts on this collective grouping; it is the aggregate you that negotiates life and either does or doesn’t contribute genes to the next generation, not your individual cells. In the Clementsian model, species interact with each other and with the environment much like cells in an organism. They form a distinct collective entity: an ecosystem that has emergent characteristics, processes, and feedbacks. Following a disturbance like a wildfire, those processes and feedbacks drive predictable patterns of ecological succession that culminate in a characteristic climax community. The climax community is stable in the sense that it will most always reconstitute itself (more or less) after perturbations. In the Clementsian model, when human management of the Arizona alfalfa field stops, it should inexorably return to its natural, stable, self-regulated state of being Sonoran Desert.
The idea of climax communities strongly influenced our approach to conservation during much of the twentieth century. We identified the important climax communities in a region and made them targets for conservation. We preserved them by preventing perturbations from happening so that the climax communities would be left alone to regulate themselves. Sometimes our perturbations were so severe that we recognized it would take a long time for the climax community to reestablish. In these cases, we tried to nudge things along, perhaps by cleaning up toxic pollution or planting pioneer species that would kick-start succession. The broad goal of this type of restoration was to help natural processes regenerate the climax community.
But ecologists gradually began to realize that the Clementsian model is mostly wrong. We have accumulated considerable evidence that ecosystems are extremely dynamic and variable. Their properties (such as what species they contain or their primary productivity) form a blurry continuum across space and time. Following a disturbance, ecosystems don’t inevitably self-organize back into a few characteristic climax communities. Instead, successional patterns are influenced by a slew of contingencies, accidents, and unique circumstances that create a diversity of outcomes. Moreover, the disturbances themselves are varied and variable, creating a temporally dynamic mosaic of different successional stages and community types across a landscape.
All the feedbacks, interactions, flows, and processes that make up ecosystems (Chap. 3). do produce some characteristic responses, predictable patterns, and some stability in the face of perturbations. For example, in Mediterranean-type ecosystems around the world, many of the plant species have evolved unique adaptations to frequent wildfire (see Fig. 3.7Figure 3.7. Convergent evolution in leaf and fruit morphology for shrub species native to four of the world’s five Mediterranean-type climates. (A) Arctostaphylos glauca from California, USA; (B) Colliguaja odorifera from central Chile; (C) Arbutus unedo from the Mediterranean basin; and (D) Acacia aneura from southwestern Australia. Their form reflects similar adaptations to dealing with prolonged drought and frequent fire. Sources: (A) Stan Shebs, https://commons.wikimedia.org/w/index.php?curid=3356203; (B) Dick Culbert, Gibsons, British Columbia, https://commons.wikimedia.org/w/index.php?curid=34451957; (C) Arbouse, Fabienkhan, https://commons.wikimedia.org/w/index.php?curid=2998058; (D) Forest and Kim Starr, https://commons.wikimedia.org/w/index.php?curid=6164977. ). Those adaptations help their populations recover from a fire, and that in turn helps drive characteristic patterns of succession. But those patterns are a reflection of natural selection acting on individuals to produce individual traits that interact with the environment. Ecosystems aren’t self-organized superorganisms, but rather a diffuse collection of individual interactions that come together more by circumstance than design.
That dynamic and circumstantial model of ecosystems has influenced nature conservation in several ways. We now recognize that disturbance and dynamic variability are inherent aspects of ecosystems that we should retain if we want to preserve their naturalness. Many of our activities during the Anthropocene have significantly altered disturbance patterns across large areas (Section 3.4). As a result, reserve managers are now in the seemingly paradoxical position of generating disturbances in order to reconstruct natural disturbance patterns. For example, fire regimes are often manipulated using approaches such as controlled burns in order to replicate what is thought to be the historical or prehuman pattern of fire for a region or ecosystem.26 That sort of active manipulation of a fundamental ecosystem process is a different approach than letting an ecosystem alone to self-regulate.
Because we now influence many of the contingencies and circumstances that shape ecosystems, we are less certain about which of the varied ecosystems that develop are natural and should be preserved. For example, we have changed many fundamental things about the environment of southern Arizona, all of which can shape the path succession takes if we were to stop management inputs to that alfalfa field. A not exhaustive list includes:
- (1) elevated soil nitrogen caused by air pollution from surrounding urbanization;27
- (2) altered rainfall patterns caused by climate change;28
- (3) degraded biological soil crusts (which alters hydrology) caused by activities such as livestock trampling,29
- (4) increased fire frequency caused by non-native grasses such as bufflegrass (Pennisetum ciliare)30
- (5) disrupted pollination and seed dispersal mutualisms caused by habitat fragmentation.31
It is therefore unlikely that whatever community that autonomously develops as a result of succession is going to closely match the ecosystem that occupied the site 100 or 200 years ago. In many parts of the Sonoran Desert, what were once diverse shrublands are gradually shifting into grasslands (Fig. 9.4), driven by the subtle nudges from a range of human alterations to the system. Once formed, these grasslands exist more or less autonomously. But can we call them Sonoran Desert? They are a unique mixture of native and non-native species, they have an historically atypical fire regime, and they provide a different set of ecosystem services compared to what we have called Sonoran Desert in the past.32
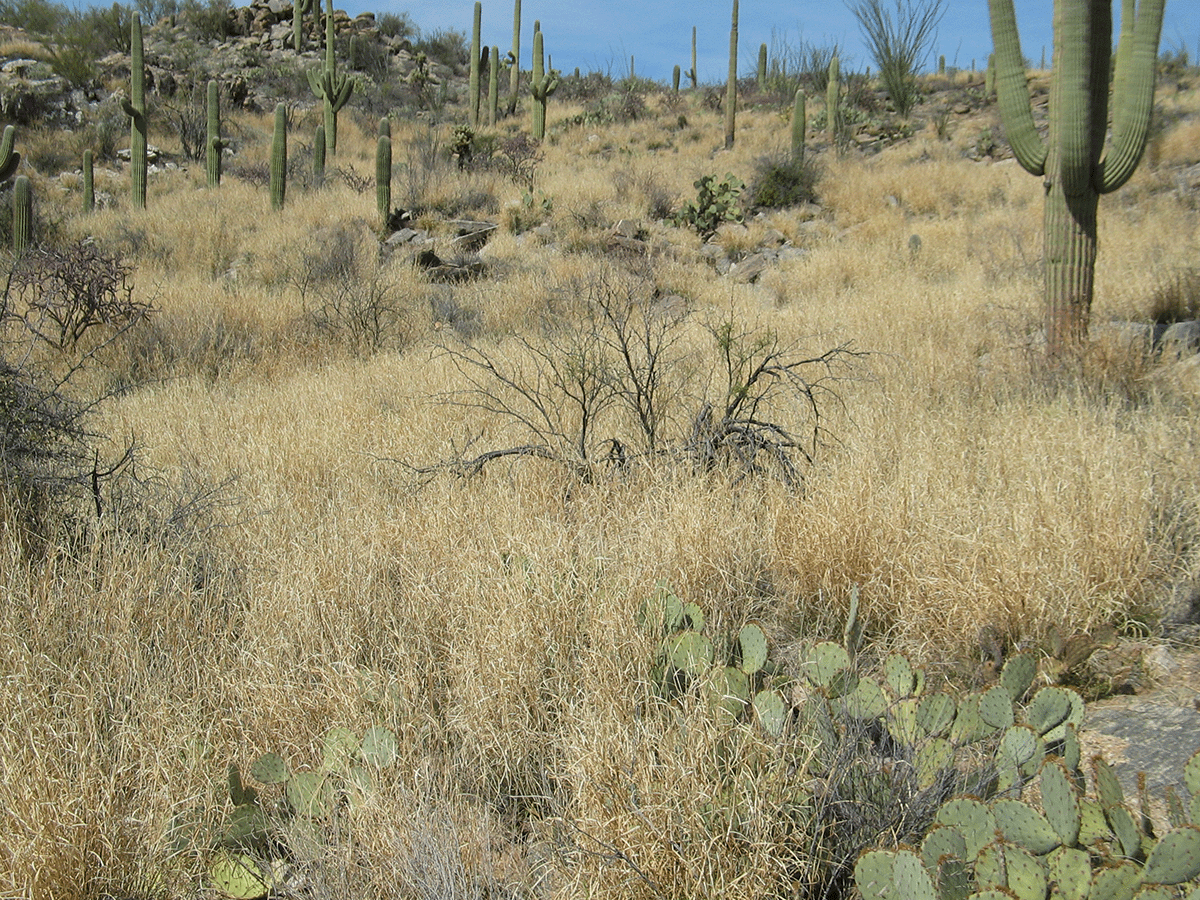
These new ecosystem configurations have been termed novel ecosystems, and we are struggling with what to make of them. On one hand, they are partly products of the myriad changes we have caused to the Earth System. On the other, they are autonomous and exist independent of our direct management. Should we treat them as unnatural or natural systems when we develop management and conservation plans? Should we leave them alone to develop as they will, or should we actively manage them to make them function like we want them to?
9.2 Ecological Restoration
Section 9.2: Ecological Restoration
As changes to the Earth System accelerated during the latter half of the twentieth century, many people argued that we needed a more comprehensive and aggressive approach to conservation. They thought that in addition to preserving pristine examples of nature and reducing the human activities that destroy nature, we should also try to actively rejuvenate human-degraded nature and even attempt to re-create destroyed nature. The scientific discipline of restoration ecology developed to figure out how best to do that. The Society for Ecological Restoration (SER) puts it this way:
Across the globe, centuries of unsustainable activities have damaged the aquatic, marine, and terrestrial environments that underpin our economies and societies and give rise to a diversity of wildlife and plants. SER is dedicated to reversing this degradation and restoring the earth for the benefit of humans and nature.33
The concept was born of a seeming contradiction: to preserve nature we might need to seed it, cultivate it, and manage it.
Both the science of restoration ecology and the practice of ecological restoration have always struggled with that existential unease. The ecological part of the term was chosen to distinguish ecological restoration from other more human-focused types of first aid that we provide to ecosystems. Examples of the latter type include replanting timber trees following a clear-cut, restocking a depleted fishery, and planting a fast-growing non-native grass to reduce erosion along a new road cut. Those manipulations are narrowly focused on achieving a few products and services we care about: the amount of harvestable timber in 30 years, the salmon catch a few seasons from now, or a reduced risk of landslide. The manipulations carried out to achieve those goals are often done with little or no regard to the broader ecosystem or to conserving biodiversity. For example, timber trees are often replanted as a monoculture to maximize the yield of marketable timber, not to maximize habitat value or biodiversity. It is restoration done primarily to provide narrow, often short-term, human goals.
In contrast, the manipulations done as part of ecological restoration are meant to focus less on direct, short-term human benefits and more on enhancing the well-being of the broader ecosystem. Goals typically include restoring habitat for native species and increasing overall levels of biodiversity. Many of the specific practices of ecological restoration such as planting native plants or removing a dam on a river are meant to kick-start autonomous ecological processes that will shape ecosystem development without human manipulation. It is restoration mainly to benefit nature, although hopefully along the way we will also improve the range of ecosystem services that nature provides us.
We face enormous technical and conceptual challenges in attempting to achieve those goals. These challenges are less about our ability to manipulate ecosystems and more about our limited understanding of how ecosystems work and how to put them back together again when we break them. In addition, the Anthropocene has increased our ambiguity about what nature is and what the goals of ecological restoration should therefore be. The mission statement of the Society for Ecological Restoration quoted above reflects that ambiguity. It spends as much time describing the benefit to humans that ecological restoration provides as it does the benefit to nature. Despite these technical and conceptual challenges, ecological restoration has had some successes and produced a number of benefits—for both nature and people. Although restoration ecology began as a subdiscipline of conservation biology, in many ways the concepts and techniques that have been developed for ecological restoration are being applied more broadly as tools for conserving and preserving biodiversity. The realization that the entire world is being altered and changed by our activities has forced us to consider using more proactive manipulations and interventions even in those parts of the world that we set aside as nature and protect from human influence.
In this section, I describe how ecological restoration is done, what potential benefits it offers, and what some of the challenges are.
Describing Damages and Identifying Causes
Like any good repair project, one of the first steps in ecological restoration is to identify how the ecosystem is damaged and what caused or is causing the damage. This is often straightforward since many of the disturbances we inflict on ecosystems are recent, acute, and caused by well-documented activities. Some examples from a long list include oil spills, forest clear-cuts, mining activities, dams, and grazing by domesticated animals. In cases such as these, we often know when the damaging activities started and how the ecosystem looked and functioned before the perturbations changed things. That allows us to more easily catalog what the damages have been and to develop plans to reverse them.
Things are not always so straightforward, however. One complication is that we often don’t have a good idea what the ecosystem looked like before our activities changed things. This can occur because the activities that triggered changes happened a long time ago, before anyone was keeping good ecological records, or because the perturbation happened in an ecosystem that we currently know little about. An example of the latter is the Deepwater Horizon oil spill that occurred off the Louisiana Coast in 2010. The wellhead blowout happened at a depth of 1,500 m, and much of the released hydrocarbons stayed in the deep ocean environment. We have a poor understanding of the deep ocean Gulf of Mexico ecosystem in general, and we had almost no ecological data from the specific area where the blowout occurred. This made it difficult to assess what damages the spill caused and to figure out what we might do to help the ecosystem recover.34
Another complication is that ecosystem degradation is often not caused by a single acute event but by multiple interacting activities that operate over different temporal and spatial scales. The various factors contributing to the degradation of coral reefs that I describe in Section 6.8 are classic examples. Some activities like coral-destroying fishing practices are local and immediate, while others such as eutrophication and ocean acidification are widespread and chronic. Still other factors such as human-caused climate change alter the timing or severity of periodic natural perturbations such as heat waves. How ecosystems respond to these mixes of perturbations is just as complex. Ecosystems can be resilient to individual perturbations but not their combined influence. In some cases, chronic perturbations reduce the ability of the ecosystem to resist or recover from future acute perturbations or increases in the level of chronic perturbations. In these cases, the ecosystem may outwardly appear to be relatively unaltered but is actually on the edge of a tipping point of radical change. Many of the world’s coral reefs are probably at such a tipping point right now. Identifying tipping points usually requires a much more detailed understanding of ecological conditions, processes, and feedbacks than we currently have for many ecosystems. In the case of coral reefs, relatively easy-to-gather metrics such as the current total abundance of coral or coral species diversity might not reflect underlying changes to the reef system that have reduced its ability to resist or recover from a future disturbance. For instance, although the adult corals on a reef may look fine, chronic water pollution may have reduced their recruitment success or increased their susceptibility to bleaching events. Measuring coral recruitment or physiological health is much more time consuming and technically challenging than simply measuring the abundance of adult coral.
The difficulties we sometimes have in assessing the overall health of ecosystems or in diagnosing the root causes of decline make it difficult to prioritize the ecosystems and locations where restoration efforts would have the biggest beneficial impact. For instance, in the case of coral reefs, the biggest return of conservation effort could result from restoring many currently modestly degraded coral reefs so that they move away from the edge of a tipping point instead of investing a lot of effort in trying to restore a few already severely degraded reefs that have crossed over a tipping point.35
Identifying a Goal
An important aspect of any repair project is describing what your goal is. A clearly defined goal helps you plan how to make the repairs, suggests what tools you will need, and sets the benchmark for deciding whether the repairs have been successful. But describing the goals for ecological restoration is often far more complicated than describing the goals for a typical repair project—although if you have done any home renovations, you might see some similarities!
There are four broad approaches to setting restoration goals. Each have advantages and pitfalls, and we often combine the different approaches. Restoration ecologists are also currently critically evaluating the usefulness and practicality of each approach as the Anthropocene progresses.
Return to the Most Recent Undisturbed Condition
A usual restoration goal is to return an ecosystem to how it looked and functioned prior to a disturbance (Fig. 9.5). In cases such as an oil spill, where the perturbation is recent, well-defined, and acute, returning to the pre-disturbance baseline can be reasonably straightforward (Fig. 9.5A). If we have ecological data about the restoration site from before the disturbance, we can use those to define success metrics. More commonly, we don’t have detailed pre-disturbance records. Instead, we typically use current conditions at undisturbed reference locations to set benchmarks. Typical criteria for choosing appropriate reference sites include:
- (1) historical evidence (e.g., pictures, land records) that the restoration site used to be ecologically similar to the reference sites
- (2) current ecological clues such as similar abiotic conditions or spatial proximity that suggest the restoration site used to resemble the reference sites
- (3) evidence that the reference site have not been affected by the disturbance that damaged the restoration site
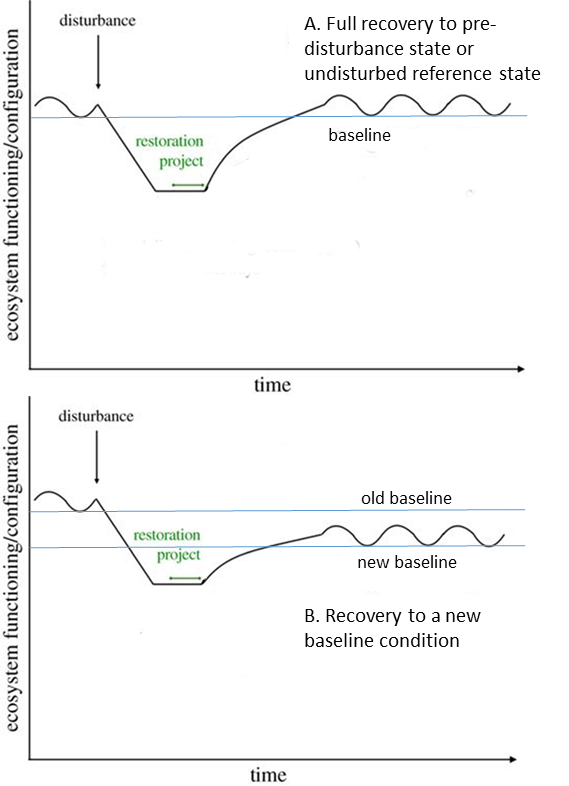
Even if we have historic information about a restoration site, it is still good practice to use contemporary reference sites to help set restoration goals. This is because any historical information we have about a site usually only depicts a narrow snapshot in time and space, yet we know that ecosystems inherently vary in both time and space. In Figure 9.5, we can see that site conditions vary before the acute disturbance and after the restoration. Restoration goals should reflect that natural level of variability. We can do that by observing how ecological traits differ among a set of reference sites and how they vary over time. Often a minimum benchmark is used to define successful restoration, such as a minimum amount of native vegetation cover. In other cases, restoring the natural range of spatial or temporal variability is a more explicit goal. In these cases, success is defined in terms of whether the restored site achieves a specific pattern of variability in conditions. For example, one of the main ways that dams cause ecological changes to aquatic ecosystems is by altering the natural variability of water flow, particularly the magnitude and timing of alternating periods of high and low water.36 A goal of river restoration is often to restore more natural flow patterns through efforts like altering the timing and amount of water releases from dams, or even removing dams altogether.37
One complication with using the recent past or nearby conditions as benchmarks is that ecosystems are rapidly changing during the Anthropocene. Reference conditions have become moving targets that are shifting outside of the range of their historical variability, creating a shifting baseline of ecological conditions (Fig. 9.5B). We are uncertain about how to deal with these shifts when setting restoration goals. In some cases, the shifting baseline can limit our imagination for what is possible. We may begin to view radically altered ecosystems as simply being the new normal. That perspective can foster complacency about addressing our negative impacts, or even a fatalism that paralyzes action on conservation and restoration. A good example is the rapidly eroding Mississippi River Delta (see the introduction to Chap. 5). The delta used to be a much more widespread expanse of tidal wetlands, forested islands, and bottomland hardwood forest bisected by an intricate network of water channels. But we have converted a large proportion of that semiterrestrial landscape into open Gulf of Mexico, primarily by walling off the wetland from its nourishing supply of Mississippi River sediment. Newly arrived residents likely see the more marine nature of the ecosystem as the normal, natural condition, even though the shift has only happened within the past 100 years or so and the causes have been far from normal or natural. Longtime residents have adapted to the new environment. For instance, the sunken former wetland makes navigating the region by boat treacherous. Captains use old navigation charts from the 1950s and earlier because they show the original navigable distributary channels. Many residents even prefer the new conditions because it makes harvesting some species such as oysters and marine fish easier.38
However, we have the technical ability to return things—more or less—to how they were. One approach is to use a large pipe to move sediment-filled water from the river onto the adjacent marsh plane (Fig. 9.6). Since the river sits above the elevation of the marsh, the pipe acts as a siphon and gravity does most of the work. These types of diversions allow us to maintain the flood control levee and a channelized river for navigation while also periodically replenishing the marsh with sediment. Only a few river diversions have been constructed so far, but it would be straightforward to construct more and significantly restore much of the original sediment distribution patterns of the delta. We haven’t done so yet because of political, social, and economic reasons, not technical ones.
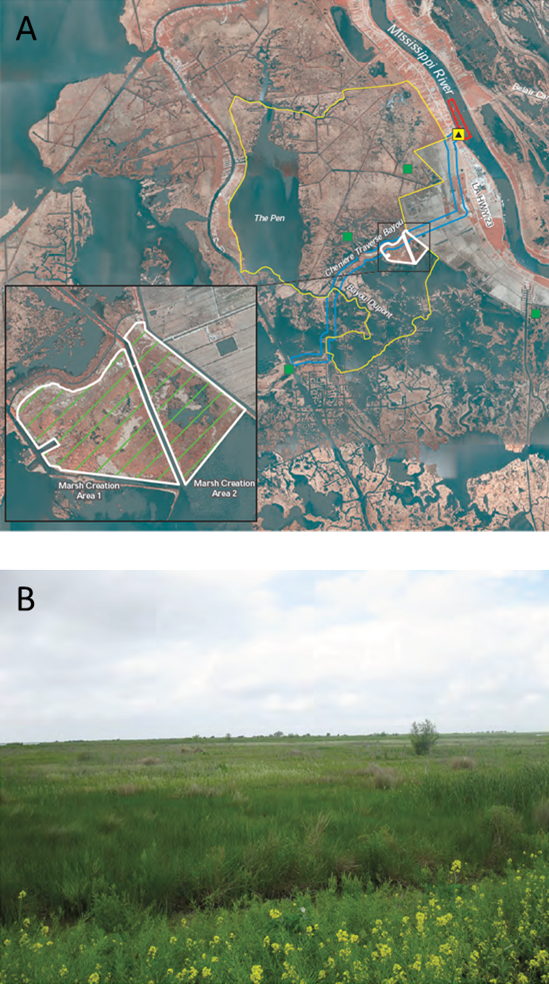
A wrinkle to the shifting baseline issue is that it can affect how we perceive which areas are most in need of restoration—and by extension who gets stuck with the bill for doing the restoration. For example, large animals (megafauna) have been particularly affected by our activities, and their abundance has been dramatically reduced or they have been extirpated from many regions. Megafauna play disproportionately large functional roles in ecosystems, so restoring their populations is often a priority restoration goal. Regions have lost their megafauna to varying degrees and at different times, however. In North America and Europe, a lot of the losses of terrestrial megafauna happened during the Late Pleistocene and Early Holocene, 50,000–7,000 years ago (see Fig. 1.5Figure 1.5. A postcard from Los Angeles, California, during the Pleistocene. None of the large animal species in the picture survived into the Holocene: Harlan’s ground sloth (right foreground), saber-toothed cat (left foreground), Merriam’s teratorn (perched on tree), and Columbian mammoth (distant background). Source: Charles R. Knight, The Jesse Earl Hyde Collection, Department of Geological Sciences, Case Western Reserve University, https://commons.wikimedia.org/w/index.php?curid=7989577. ). In other regions like Africa and Southeast Asia, megafauna losses have continued into the present, with particularly steep recent declines. If we use the recent past as a benchmark, Asia and Africa are clear high-priority areas for megafauna restoration. Species such as tigers, elephants, and gorillas have become symbols in global conservation campaigns. But Europe and North America have experienced just as significant losses of megafauna if we take a longer time perspective. Is it fair to place all the costs of restoring megafauna on Asia and Africa, particularly since countries in those regions often have less financial and social capacity to do restoration than countries in North America and Europe?39 One solution is to share the costs of doing restoration more broadly. Another is to broaden where we think megafauna restoration is needed by taking a longer time perspective.
Return to a More Distant Past Condition
One way to avoid the muddle of current human disturbances is to model goals after conditions in the more distant past—when our influence on ecosystems was presumably less. But choosing how far to look back is inherently subjective, and like anything subjective, the choice is rife with our biases. In North America, for example, a common goal has been to restore ecosystems to conditions that we think existed prior to European colonization. One rationale for using that as a benchmark is that the arrival of Europeans catalyzed a number of processes such as the spread of non-native species and changes in farming and other land use practices that would eventually transform ecosystems across the continent. But another implicit rationale was that most North American ecologists—who were overwhelmingly of European decent—considered pre-European North America to be an archetype of pristine nature. They were largely ignorant (often willfully so) of the degree to which the Indigenous peoples of the continent had already transformed it. For instance, the flower meadows of the Central Valley of California that John Muir described (see the introduction to Chap. 7) were likely partially maintained by prescribed burns practiced by Indigenous people.40
Another complication with looking to the distant past for restoration goals is that in most practical senses, the past is impossible to completely re-create. For example, ecologists have proposed restoring the Pleistocene megafuana of North America. We can’t restore the extinct species, but we can restore some of the functions those species provided by introducing non-native taxa that are functional equivalents.41 But reintroducing even non-native large mammals is now no easy thing, particularly in landscapes that are far removed from the last time they supported an abundance of large animals. Many of the forces that caused large mammals to go extinct are still around and intensifying. We can’t simply re-create past ecosystems. We have to design them to fit the current conditions and constraints.
Make Ecosystem Resilience the Goal
One approach to dealing with the fact that current conditions are rapidly changing is to future-proof restoration by setting goals based on predicted trajectories of ecosystem change instead of on past or current reference conditions.42 An example is replanting a disturbed forest using a mix of tree species that we think will be well suited to the climate that the location is expected to experience in 50 years (when the trees are mature), not the species mix that was present at the location prior to the disturbance.43 An extension of this idea is to make ecosystem resilience the primary goal of restoration and (more broadly) conservation. Instead of setting goals based primarily on the unique traits of ecosystems that exist at particular points in space or time (i.e., reference benchmarks), we can aim to restore the processes that make ecosystems resilient to disturbances and changing conditions.
That is easier said than done, partly because we are uncertain about what those key processes are. We are, however, beginning to develop a better understanding of the processes that foster resilience in specific ecosystems. For example, in coral reefs, factors that promote resilience include high levels of functional and habitat biodiversity, high levels of dispersal and gene flow between reefs, and reduced levels of chronic stresses such as polluted water.44 These concepts can be used to set specific targets for restoration and management that are not tied directly to past or current reference site conditions. Because many of the processes that influence ecosystem resilience operate across large spatial scales, resilience-oriented goals are typically defined in terms of emergent properties of whole landscapes, not just conditions at specific sites. For example, one goal for restoration could be to reconnect currently isolated habitat fragments in a landscape through techniques such as creating habitat corridors. Enhancing the habitat connectivity of a landscape potentially improves a number of factors related to ecosystem resilience, such as preventing genetic inbreeding of isolated populations and allowing local populations to recover demographically through dispersal from other populations.45 We can also design ecosystem resilience to account for expected future stresses. For instance, we can plan the placement and design of habitat corridors in ways that will help populations adjust their ranges in response to climate change. I discuss these climate corridors as well as other approaches for enhancing the resilience of ecosystems in Chapter 10.
Developing resilience-based goals is also helped by our increasing ability to collect data at landscape scales, such as from satellite-based environmental sensors. We can integrate those data using tools such as a geographic information system (GIS) to create more comprehensive pictures of ecosystem conditions. We can use this information to parametrize models that predict how ecological conditions will change in the future, in much the same way as we develop climate prediction models. An example comes from a project that tried to understand how the landscape of the Willamette Valley in Oregon might look by the middle of the twenty-first century. The researchers compiled a wide range of data on factors that influence land use patterns, including human demographic changes and the relationship between economic activity and the demand for housing and agricultural land. They also compiled a range of ecological data that characterized the past and present ecosystem types and land use patterns across the Willamette Valley. They then used all that information to parameterize models that predicted how land use patterns would change over the next several decades under different assumptions. One scenario assumed current land use polices would continue, another assumed that conservation restrictions on development would be loosened, and a third assumed conservation policies would be strengthened. The resulting maps can help develop restoration plans that are not only based on current conditions but also take into account likely future trajectories of change caused by factors such as shifting human demands for land or the impact of climate change (Fig. 9.7).
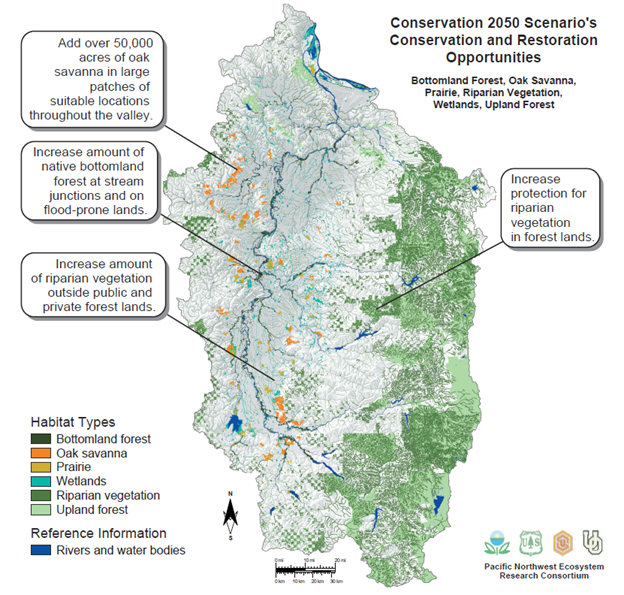
Adaptively Manage Functional Goals
As mentioned above, ecological restoration originally developed as an alternative to the utilitarian and human-centric way in which we often managed ecosystems. As a result, the goals for ecological restoration often explicitly excluded humans: the ultimate goal was a self-sustaining autonomous ecosystem free from human meddling. Early restoration goals often downplayed any direct benefits the restored ecosystems might have for humans such as food or harvestable timber; the prime benefactor was intended to be nature itself. But we are increasingly developing restoration goals that explicitly include people as integral and active participants, that is, as social-ecological systems (see also Section 8.5).
One way this is manifesting itself is that restoration and broader conservation are beginning to incorporate chronic human interactions and management as acceptable and even integral aspects of restoration goals. A good example is the increasing appreciation of the long-term historical ecosystem management practiced by local or Indigenous peoples. For instance, the Western Desert ecosystem of Australia has been strongly shaped by the activities of its first human inhabitants. This management includes prescribed fire, selective hunting, dispersing preferred species, and disturbing soil to create habitat for species. In fact, one way in which subsequent European colonists altered the Australian Western Desert was by removing Indigenous communities and halting their traditional land management activities. One goal (and also a tool) for restoring the degraded Western Desert could be to restore traditional land management.46
Restoration goals are also beginning to be framed in terms of ecosystem services—the benefits we get from ecosystems. For example, in coastal Thailand, an explicit goal for restoring shrimp farms back into mangrove forest is to increase the storm protection and fishing services the mangrove forests provide to local communities (see Fig. 2.19Figure 2.19. In Thailand, coastal communities often convert mangroves into shrimp farms, providing a direct economic benefit to communities. But converting mangroves to shrimp farms involves a trade-off between the value of the shrimp farms and the loss of value from the mangroves. The net return from shrimp farms is linearly related to the proportion of the coast in shrimp farms (a negative linear relationship with the proportion in mangroves). In contrast, the net return from mangroves is an asymptotic relationship with mangrove area. As a result, the maximum net total return from both shrimp farms and mangroves is achieved when there is a mix of about 80% mangroves and 20% shrimp farms (indicated by the dotted lines). Source: drawn from data reported by Barbier et al. (2008). ). Framing goals in terms of ecosystem services can be an effective way to invest local communities in restoring and preserving ecosystems.47 But framing goals in this way can also—at least to some people—begin to sound eerily similar to the human-focused utilitarian management that restoration ecology emerged to counter. If we restore a mangrove forest primarily to provide ecosystem service benefits to local communities, are we less worried about making sure the end product also provides broad benefits to nature? In concept at least, benefitting humans and nature are not mutually exclusive goals. But as the Anthropocene illustrates, they are also not inexorably linked. Still, the hope is that by incorporating human needs and desires into restoration goals, we can better align our own well-being with that of nature.
Moving toward the Goal
We are developing an increasingly sophisticated set of techniques and approaches for achieving restoration goals. These tools integrate ecological information about how specific ecosystems function with our immense (and still increasing) technical prowess at manipulating ecosystems. Approaches are as varied as the ecosystems we are attempting to restore, our goals, and the sociopolitical contexts of each project. I won’t attempt to detail any of that complexity. Instead, I’ll provide a broad overview of the types of approaches we typically take. If you are interested in delving a little bit deeper into the topic, see the additional resources.
Getting an ecosystem to move toward restoration goals involves two distinct steps. The first is stopping whatever perturbations degraded (or are still degrading) the ecosystem. The second is imposing new perturbations to nudge (or shove) the ecosystem toward the goals. The first step is often straightforward, such as in the case of an acute accident like an oil spill. Also, stopping the disturbance may be all that is needed to allow the ecosystem to recover on its own so that we don’t even need a step two. A meta-analysis that analyzed ecosystem recovery from large-scale disturbances such as oil spills found that active restoration often did not result in faster or more complete recovery than simply ending the disturbances,48 although the results of that study are sensitive to exactly how we define recovery.49
In cases where perturbations are many, chronic, and interacting, stopping them—or at least stopping all of them—can be difficult to impossible. As I describe above, one way to address that issue is by adjusting how we frame restoration goals—for instance, by making ecosystem resilience a primary goal. Another way is to use ongoing management to counteract the effects of chronic perturbations. An example comes from the Sisyphean effort to control Himalayan blackberry (Rubus armeniacus) at restoration sites in the US Pacific Northwest. Himalayan blackberry is a widespread invasive species in the region. Its success is partly related to the fact that the birds and small mammals who love to eat blackberries disperse blackberry seeds across the landscape in their feces. Given how abundant and widespread Himalayan blackberry now is, that rain of bird- and mammal-driven seeds is impossible to stop. Some strategies can help native vegetation resist Himalayan blackberry establishment, but none of them are completely effective.50 Riparian zones are particularly vulnerable since their narrow, relatively open tree canopies don’t provide much resistance in the form of shade, which blackberries don’t particularly like. As a result, almost any riparian restoration in western Oregon and Washington will sooner or later be colonized by Himalayan blackberry.
Riparian restoration goals in the region now accept that some low level of Himalayan blackberry will likely always be present. Many restoration plans take a pragmatic integrative pest management approach and conduct continual, periodic weeding to keep Himalayan blackberry at levels low enough to ensure other goals are met, such as maintaining native understory plant diversity. Whether we should call a restoration successful even if it requires in-perpetuity management like weeding is another of the Anthropocene-driven conundrums that have spurred lively debates among restoration professionals in recent years. After all, isn’t one of the main distinctions between ecological restoration and other forms of ecosystem management such as agriculture the idea that the end goal is a self-sustaining autonomous ecosystem, not one in need of constant human intervention? Invasive species management in general involves a range of other related conundrums and issues. I explore these in more detail in the next section.
Weeding is just one of the diverse range of techniques we can use to push ecosystems toward restoration goals. These techniques target or utilize all the main ecosystem components (Table 9.1). Many of the specific techniques and tools that we employ—such as culling undesirable species, adjusting soil nutrient levels, rearranging topography, and mass rearing of nursery stock—are the same ones we use to create and manage our domesticated landscapes.
Restorative Practices and Their Targets and Utilizations | ||
---|---|---|
Ecosystem Component | Common Activities | Examples |
Abiotic Conditions |
|
![]() Spreading dredged sand to adjust tidal elevation on Seatuck National Wildlife Refuge. |
Biotic Conditions |
|
![]() A pack of reintroduced wolves in Yellowstone National Park.[/p] |
Flow of Energy and Materials |
|
![]() Removal of the Marmot Dam, Sandy River, Oregon[/p] |
Biophysical Feedback |
|
![]() Efforts to eradicate non-native beavers from Tierra del Fuego. |
Restorative Practices and Their Targets and Utilizations | ||
---|---|---|
Ecosystem Component | Common Activities | Examples |
Abiotic Conditions |
|
![]() Spreading dredged sand to adjust tidal elevation on Seatuck National Wildlife Refuge. |
Biotic Conditions |
|
![]() A pack of reintroduced wolves in Yellowstone National Park. |
Flow of Energy and Materials |
|
![]() Removal of the Marmot Dam, Sandy River, Oregon |
Biophysical Feedback |
|
![]() Efforts to eradicate non-native beavers from Tierra del Fuego. |
We must adapt these techniques and tools in ways that reflect the distinct goals we have for ecological restoration. Broadly, there are three main concepts involved in restoration efforts.
An Emphasis on Native and Non-Domesticated Biodiversity Patterns
A usual restoration goal is to create patterns of genetic, species, functional, and habitat diversity that reflect the evolutionary history and current ecological conditions of a site. That is typically not the goal when we manage our domesticated ecosystems. Instead, the biodiversity of domesticated ecosystems is dominated by a few forms that humans select and modify to produce a few traits and functions we want. We also create novel amalgamations of biodiversity that largely ignore and are divorced from local evolutionary histories or inherent environmental conditions. In contrast, being mindful about how ecology and evolution shape biodiversity patterns is a central aspect of restoration ecology. For example, blue-bunch wheatgrass (Pseudoroegneria spicata) grows throughout the western United States. Across its large and ecologically diverse range, blue-bunch wheatgrass populations have evolved into a number of genetically and functionally distinct forms. Some of the differences among populations are ecotypes—the differences reflect adaptations to local environmental conditions such as annual rainfall and temperature. A map of these ecotype differences helps restoration managers match blue-bunch wheatgrass varieties to their local site conditions (Fig. 9.8). In aggregate, using locally adapted ecotypes at individual restoration sites also helps to conserve the full genetic and functional diversity across the entire regional range of blue-bunch wheatgrass.
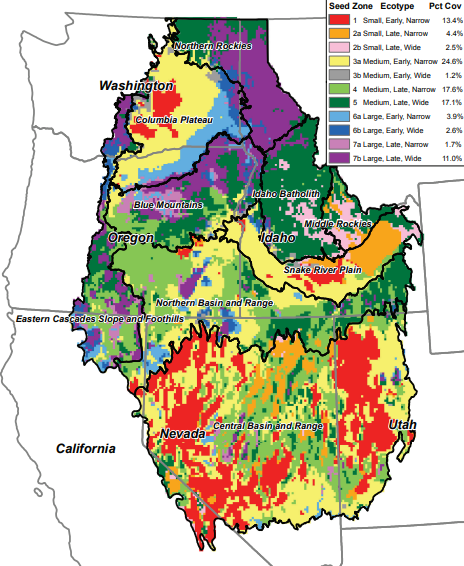
environmental conditions. Each ecotype has unique functional characteristics. The ecotype descriptions in the legend are based on these functional differences and refer to plant size, flowering date, and leaf width. “Pct Cov” is the average percentage cover (or abundance) of bluebunch wheatgrass cover. Source: Koch et al., (2015).
But of course, restoration managers need more than a map—they need actual individuals, usually in large quantities. Here again, the process of rearing individuals for restoration is distinct from the approach we take for agricultural or ornamental crops. We typically mass-raise domesticated species as genetically uniform (often identical) individuals from a few selections or human-modified forms. For instance, many of the plants available at nurseries and garden centers have been raised via asexual propagation methods. Whether purchased in Los Angeles, London, or Beijing, the plants are genetically identical. In sharp contrast, when we propagate plants for use in ecological restoration, we try to retain as much of the natural genetic and functional diversity present in wild populations as we can. For smaller projects, seeds (or other propagules) can be collected directly from the wild. Care is taken to collect seeds from an appropriate sample of individuals and populations within seed zones to ensure that as much existing genetic diversity is represented in the sample as possible. For larger projects, we need to mass-rear plants in nurseries. But the propagation process can distort genetic composition, so that even if we use starting stock from wild-collected seed, the resulting nursery populations can still significantly diverge genetically from wild populations.51 For instance, nurseries typically have different abiotic conditions than those in the field, and the unique environment can drive selection that alters genetic composition. Nursery-reared populations can even develop unique suites of functional traits relative to wild-type populations. We are developing some solutions. For instance, we can use genetic tools to test for the possibility of inadvertent selection. I describe some other approaches in Section 10.1.
An Emphasis on Natural Spatial Patterns and Heterogeneity
Ecosystems have characteristic spatial patterns of individuals, habitats, and abiotic conditions. Restoring these patterns is often a principal goal of ecological restoration. The opposite is the case in our domesticated ecosystems, where we tend to homogenize conditions or create our own novel patterns. Restoring natural patterns of environmental variability is often a critical first step in restoring biodiversity. In many wetlands such as salt marshes, for example, tiny (to us) differences in elevation can create big differences in ecologically important conditions such as how long plant roots are waterlogged, where seeds are deposited, and the temperature and salinity that aquatic organisms must deal with.52 As a result, the topography of wetlands—specifically the spatial variability of the topography—has a big influence on biodiversity. In wetland restoration, earth-moving equipment can help restore natural topographic variability such as tidal channels, gently sloping or undulating areas, and scattered mounds and hummocks;53 see Table 9.1.
Spatial patterns also influence many of the autonomous ecological processes that restoration hopes to restart. These include pollination, seed dispersal, gene flow, competition, and facilitation. A number of restoration techniques are aimed at restoring these spatially dependent processes. For example, how individual plants are arranged when they are planted out at a restoration site can influence how well the plants are pollinated, how well their seeds are effectively dispersed, the degree to which they resist invasion by non-native species, and how well the vegetation functions as habitat for other organisms.54
An Emphasis on Natural Disturbance and Ecological Flow Patterns
A common way in which we degrade natural ecosystems as well as manage our domesticated ones is by manipulating disturbances such as fire and the flows of energy and materials such as biomass and water. In fact, just stopping the activities that are altering natural disturbance and flow patterns is a powerful tool for ecological restoration. Examples include removing a dam (Table 9.1), removing domesticated grazing animals that are causing soil disturbance and appropriating primary productivity, regulating hunting or fishing, and ceasing fire suppression activities.
In other circumstances, active management can be used to re-create what we think are more natural disturbance or flow regimes. But determining what natural disturbance patterns are can sometimes be difficult. In the United States, for example, research natural areas are a network of federally protected lands designed to preserve natural, undisturbed examples of the nation’s ecosystems. In part, they are meant to provide a baseline for monitoring ecosystem change and to provide a reference for restoration. The core management philosophy of these areas has been to leave them alone and to let natural processes shape their biodiversity patterns. Shielding these sites from unwanted human caused disturbances has become increasingly difficult, however. Human-altered fire frequency is one such disturbance. In California, 76% of research natural areas currently experience a fire frequency that is significantly different from their estimated pre-1850 frequency. Of these, 87% are burning less frequently and 13% are burning more frequently than they did prior to 1850.55If a goal is to conserve the biodiversity patterns that have evolved in response to the pre-1850 fire frequency, ongoing active management such as prescribed burns, local fire suppression, or forest thinning (to mimic an effect of fire) will be needed. But more fundamentally, it is likely that in many places the pre-1850 fire regime was not natural but the result of Indigenous management. Should that human-regulated fire regime be our restoration goal?
Assessing Success
Like any repair project, we need to have an objective way of deciding whether we have met restoration goals or we still have work to do. Basically, how do we translate the conceptual goals outlined in Figure 9.5 into concrete metrics we can measure and assess? There are myriad metrics related to the different ecosystem components and restoration approaches outlined in Table 9.1. On a practical level, most restoration projects only have the money and resources to assess a few metrics, and then often only over a short period. Consequently, a lot of the science of restoration ecology has focused on trying to identify key indicators related to specific restoration goals and that are relevant for specific ecosystems. Two important criteria for these indicators are that they be relatively easy to measure and that they be correlated in some way with at least one of the restoration goals. Indicators can be developed from any ecosystem component or process. Just a few examples from a potentially unlimited list include soil nutrient levels, the abundance or recruitment success of a particular species, the taxonomic richness of a particular group such as plants, net primary productivity, and the amount of tree cover. Among the most commonly used indicators are those based on the components of biodiversity. This is partly because increasing biodiversity or altering patterns of biodiversity are often the main proximate goals for restoration. But comprehensively assessing biodiversity is difficult and time consuming. Instead, a narrow aspect of biodiversity is usually chosen to serve as a proxy measure for broader biodiversity goals. For instance, plant species richness is a common restoration metric because it is relatively easy to measure, and it is known to correlate with other aspects of biodiversity such as insect functional diversity.
Practical constraints of time and money have also spurred the search for indicator species, whose characteristics relate in some quantifiable way to broader ecosystem characteristics. Measuring the traits of a single species is a lot easier than trying to assess all the intricate parts of complex ecosystems. Good indicator species are ones whose traits (like abundance or physiological condition) are sensitive to broader ecosystem conditions or to stresses like pollutants that are the focus of restoration goals. Other good indicator species are ones that strongly influence ecosystem conditions such as keystone species and strong ecosystem engineers whose presence and abundance have a disproportionate influence on ecosystem properties. For example, eelgrasses (Zostera spp.) are critical parts of temperate nearshore marine ecosystems. Their ecosystem engineering creates physical habitat and their primary productivity feeds a complex trophic network, both of which support a wide range of other organisms.56 Eelgrass abundance and primary productivity are therefore commonly used as success metrics for the restoration of temperate nearshore habitats.57
Despite considerable progress in identifying important indicators of restoration success, the complexity of ecosystems, as well as their spatial and temporal variability, still makes evaluating success difficult and often unsatisfying. A good example is the recovery of Prince William Sound, Alaska, following the Exxon Valdez oil spill in 1989. After the spill, restoration efforts had a clear goal: to limit the acute and long-term damage to wildlife caused by the oil. To do this, a variety of techniques were employed to capture, immobilize, or disperse the spilled oil. Oiled wildlife were also captured, rehabilitated, and released. Undoubtedly, those efforts had some benefits, but just how beneficial and whether they appreciably enhanced ecosystem recovery in any meaningful way were less clear.58 Nearly 30 years after the spill, deciding whether the Prince William Sound ecosystem has recovered is nuanced and complicated. Populations of some species such as glaucous winged gulls and bald eagles recovered within a year or two after the spill, while populations of other species such as sea otters were still suffering chronic effects even decades later. Populations of still other species such as Pacific herring suffered steep declines in the years after the spill, but the causes seem to be the result of factors not related to the spill, such as natural abiotic variability, complex food web dynamics, and other non-spill human factors.59
In addition to the inherent complexity and variability of ecosystems, the rapidly shifting baseline conditions occurring during the Anthropocene make it even harder to assess whether restoration has been a success. More philosophically—although perhaps no less practically—can we say that we have successfully restored an ecosystem from an oil spill or overgrazing if climate change has drastically changed the system in other ways? Also, the various chronic disturbances of the Anthropocene have pushed many ecosystems near the edge of a tipping point. An acute disturbance can push a system over the edge, resulting in far more profound changes that are difficult to recover from. In these cases, restoration is more about managing the chronic changes than about repairing the immediate damage caused by the acute disturbance.
An example comes from another oil spill, that of the Deepwater Horizon in the Gulf of Mexico in 2010. Some of the spilled oil coated and killed salt marsh vegetation (Fig. 9.9), but in general, salt marsh vegetation is resilient to this type of damage, and it usually quickly recovers. Moreover, the vegetation helps protect the broader ecosystem by trapping and localizing much of the oil along the outer seaward edge of the salt marsh. But salt marshes in this region have been rapidly eroding over the past century as a result of myriad chronic human stresses, including climate change. This has made them highly vulnerable to periodic acute stresses like oil spills. In Louisiana, salt marsh edges intercepted floating oil, sparing the rest of the marsh from damage. The protection was pyrrhic, however. The sacrificial death of vegetation along the edge caused erosion rates of oiled marshes to more than double relative to non-oiled marshes.60 Efforts to restore the damaged edges by replanting failed as the transplants were quickly washed away. The oil spill interacted with the already high background rate of salt marsh erosion to trigger a cascading feedback of erosion that completely negated the mechanisms that had previously made salt marshes resilient to oil spills. Restoring Gulf Coast salt marshes after an oil spill is now more dependent on mitigating the broader factors that contribute to wetland loss, such as restricted sediment supply and climate change, than it is about cleaning up the oil and replanting salt marsh vegetation.
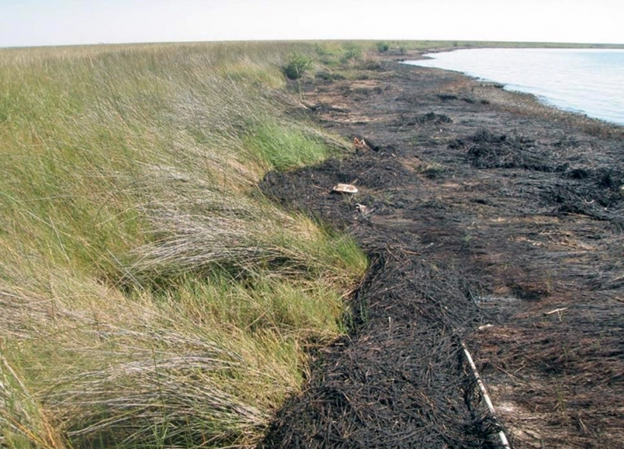
9.3 Invasive Population Management
Section 9.3: Invasive Population Management
A small subset of introduced non-native populations become self-sustaining, numerically dominant, and spread widely in their new range. In this book, I refer to this subset as invasive populations (see Table 6.4 for a summary of the confusing terminology). Managing the spread and impact of invasive populations has become a significant part of conservation biology and restoration ecology, as well as how we manage our domesticated ecosystems. This is because the ecological, economic, and human impacts associated with these populations are substantial. Our movement of species across biogeographic boundaries is one of the main ways that we are changing Earth’s biodiversity patterns and, at least on some scales, contributing to overall biodiversity loss (Section 6.5). Those biodiversity changes have negatively altered the provisioning of ecosystem services in a variety of ways, such as reduced output from fewer fisheries,61 altered fire patterns,62 and increased water scarcity.63 In addition, some translocated species cause serious problems in our agricultural and urban ecosystems, such as crop losses,64 and the spread of human diseases.65 Almost all of these impacts have direct economic costs. For example, in 2017, the economic cost inflicted by just five introduced insect pests on smallholder farmers in just six African countries was estimated to be $0.9 to $1.1 billion per year.66
A number of factors make this management difficult and at times controversial. Efforts to limit species movements or to mitigate the impacts of the introduced populations that become invasive are technically challenging and economically costly. The tools used to reduce invasive populations such as pesticides can themselves cause negative consequences for ecosystems. In addition, removing invasive populations can trigger complex ecosystem feedbacks that are difficult to predict and that often produce undesirable outcomes, making it difficult to evaluate the net effect that invasive population management has on ecosystems. Moreover, how we value and prioritize the various functions and services that ecosystems provide (and therefore the net value of managing invasive populations) varies widely across human cultures and socioeconomic contexts. The changing baselines in ecosystem conditions caused by the other drivers of the Anthropocene, such as climate change, can make returning to conditions prior to species introductions impossible. Finally, in addition to the technical challenges, invasive population management also involves philosophical questions about our relationship with nature. All of that makes it difficult to implement the best management and to assess whether a specific management action will produce more net benefits than costs.
In this section, I outline the main approaches we have developed for managing the spread and impact of invasive populations along with some of the challenges, uncertainties, and controversies related to this management.
Reducing the Movement of Species
One prong of our management effort is to prevent the introduction of potentially damaging species in the first place. The main approach to this effort involves identifying species that pose a risk of causing problems if they are moved into new biogeographic regions, attempting to prevent populations of those species from being moved, and as a last resort intercepting them while they are in transit along trade and transportation networks. Assessing invasion risk is inherently difficult because the degree to which introduced populations become invasive depends on the complex interaction between the traits of the introduced populations, the traits of the recipient ecosystems, and our own actions that change those first two things. We have developed a few tools that assess the risks that populations of a species will be introduced to a new range, that the populations will become established in the new range, and that they develop into invasive population. Table 9.2 is a generalized example of a common risk assessment rubric. While these approaches have been somewhat successful, their ability to proactively predict invasion risk is still limited.67 We still assess risk based largely on the past reputation of a species and our historical patterns of use and transport—that is, whether populations have already been introduced someplace and have caused problems.
Risk of Invasive Populations Rubric | |
---|---|
Risk Category | Assessment |
Likelihood populations of a species will be introduced to a region |
|
Likelihood introduced populations will survive and establish in the new range |
|
Likelihood introduced populations will become invasive |
|
Risk of Invasive Populations Rubric | |
---|---|
Risk Category | Assessment |
Likelihood populations of a species will be introduced to a region |
|
Likelihood introduced populations will survive and establish in the new range |
|
Likelihood introduced populations will become invasive |
|
Many countries have regulations banning the trade of species with known or suspected risks. Countries also have quarantine and inspection regimes designed to intercept the illegal or unintentional importation of risky species. Industries that trade in biological commodities have adopted rules and best practices designed to minimize the chance that unwanted species get unintentionally transported along with the traded commodity. These techniques can also be used to slow the spatial spread of populations that have already been introduced to a region. For instance, after populations of emerald ash borer (Agrilus planipennis) were first detected in Michigan in 2002, a series of quarantine zones were established that restricted the movement of wood in an attempt to prevent the beetle’s spread—not successfully, as it turned out.68
Prevention efforts have had some success, but preventing unwanted introductions has become increasingly difficult as our societies become ever more intimately interconnected.69 Not only is the volume of trade increasing, but also the geographic sources and destinations of trade have been expanding.70 Another complication is that the decision to implement restrictions on the trade of organisms is always a balance between the benefit of reducing the risk of species invasion against the costs of the regulations.
A good example of this balancing act is the trade in ornamental plants. The global nursery trade is a multibillion-dollar industry that not only generates a considerable amount of economic activity but also supplies the plants that provide important ecosystem services in our domesticated landscapes. Although only a small percentage of ornamental species ever move beyond domesticated ecosystems to cause problems, the sheer volume and global reach of the nursery trade make it the main source of naturalized and invasive plant populations.71 Many of the regulations and restrictions on species movements mentioned above have been adopted by or imposed on the nursery industry. But these regulations and restrictions can cause tangible economic harm or limit the options we have in designing our urban landscapes. For example, Norway maple (Acer platanoides) is a common ornamental tree in part because it tolerates harsh urban conditions such as air pollution. In North America, however, it has spread into natural areas where it suppresses a range of native species, including dominant trees such as sugar maple (Acer saccharum).72 Documenting these ecological impacts and risks has been a slow process, in part because the impacts play out slowly over the decadal timescales of forest generations. In contrast, the economic and other costs of restricting a useful species for urban environments are much clearer to demonstrate, which has contributed to a long and sometimes contentious process of developing regulations and best practices for the use of the species.
A complementary approach to banning or restricting the movement of potentially invasive ornamental species is to use species or cultivars that pose less of an invasive risk in the first place. There is great potential in this approach, if for no other reason than we haven’t usually chosen ornamental plants based on their risk of invasiveness. For example, a number of distinct cultivars of Norway maple have been developed largely for aesthetic traits or other traits related to their use in urban landscapes. Many of these forms also differ in traits such as seed production that likely contribute to differences in their relative invasive risk.73 The nursery industry is beginning to market plants based on their low invasive potential, and ornamental plant breeders are beginning to breed for traits such as sterility that contribute to reduced invasive risk.74.
Population Regulation
Nearly all the ways that introduced populations exert their influence on ecosystems (e.g., competition, predation, ecosystem engineering) depend to varying degrees on their local abundance. The introduced populations that exert large impacts often do so in part because their populations become unusually large or dense. One explanation for why this occurs is the enemy release hypothesis.
It is thought that introduced non-native populations typically leave behind most of the coevolved predators, competitors, and pathogens that had regulated their abundance back in their native ranges. In some cases, the new suite of enemies that the introduced populations encounter are not nearly as effective population controls as the old ones. In the most extreme cases, such as when a species is introduced to a remote island, the new ecosystem may lack predators or strong competitors of the introduced populations altogether. This has been the case for many small mammals, such as domesticated cats introduced to oceanic islands.75 An example comes from Marion Island, a remote island in the sub-Antarctic Indian Ocean. South Africa established a research station on the island in 1948 and with the people came 5 domestic cats. Without any predators and with abundant native and nearly defenseless seabirds as easy snacks, the cat population exploded to more than 2,000 in just 25 years. In the late 1970s, there were nearly 14 cats per km2 in the coastal zone of the island that killed nearly half a million diving petrels (Pelecanoididae) per year.76
Restoring some level of population regulation is therefore an important approach to invasive population management. This typically involves using the range of tools that we have developed against pests in our domesticated ecosystems such as chemical pesticides, physical weeding, trapping, fire, and biological control. But invasive population management often involves unique challenges. When invasive populations are in domesticated ecosystems such as farms, the main challenge is usually that existing approaches that have been developed for more familiar pests are ineffective. For instance, when populations of spotted wing drosophila (Drosophila suzukii) were introduced to the Americas and Europe from Asia, they inflicted significant economic damage on fruit growers. This is in part because, unlike other Drosophila species, D. suzukii can oviposit into young, still developing fruit, causing major crop losses. Because D. suzukii had not been a major pest in its native range, there was not much existing information on how to manage it as a pest.77 Faced with a dire situation, growers resorted to using generic approaches such as broad-spectrum pesticides that have significant nontarget impacts.78
Managing invasive populations in wildland or restoration contexts comes with its own unique challenges. A fundamental issue is that wildlands are more biologically diverse and spatially complex than domesticated ecosystems such as farms, and that can make deploying a particular tool prohibitively costly or not technically feasible. It also makes it even more challenging to limit unwanted negative effects from the management. For example, while tillage is an approach to weed control in agroecosystems, using it in most wildland settings isn’t feasible or can result in unacceptable impacts such as high erosion. Cost is another significant constraint. The large spatial scales as well as the logistical complexity of working in wildlands can make the financial cost of deploying effective population regulation prohibitive.
Even so, some tools are particularly suited to invasive population management. One of these is biological control, especially the form of it referred to as classical biocontrol. This involves reuniting introduced populations with some of the coevolved natural enemies from the native range of the species. If natural enemies are chosen that are narrow specialists of the target species, it should restore effective regulation to introduced populations with minimum risk to nontarget organisms.79 By some measures we have done a good job at that, at least in recent years. Between 1795 and 2008, 13% of the 457 biological control agents intentionally released to control invasive weeds had been recorded attacking nontarget species. But most of those cases of nontarget effects were for agents that were released using poor screening protocols or no screening at all. The incidence of nontarget effects has declined over time as we developed better protocols and safeguards, and there have been only a handful of true surprises where a carefully screened biocontrol organism caused unexpected nontarget impacts.80 Still, finding appropriate natural enemies and screening them for safety is time consuming and costly, just two factors that limit its more widespread use.
Other approaches are more adaptable to wildland and restoration contexts than they are to domesticated ecosystems. One broad approach is to restore physical conditions to an undisturbed state. Many species that frequently form invasive populations have adaptations to physical conditions that we create, such as high nutrient levels or frequent disturbance. In fact, a range of species have benefitted from the changes associated with the Anthropocene (see Section 6.1). That may be one reason why populations of these species are often successful when we introduce them to new regions. For example, many grass species are particularly suited to high soil nitrogen, and our eutrophication of landscapes has contributed to their spread and success in many regions where they have been introduced. A specific example is buffelgrass, whose spread across the warm deserts of North America (Fig. 9.4) has likely been partly helped by nitrogen deposition from air pollution.81 Stopping the disturbances or restoring pre-disturbance conditions can therefore be an effective way to regulate non-native populations. For instance, adding high-carbon soil amendments such as sawdust or biochar to a restoration site can feed a spurt of microbial biomass that immobilizes plant available nitrogen. Lower nitrogen availability can in turn allow native populations to gain the upper hand in competition with non-native populations.82
Integrated Conservation Management
Managing invasive populations has become a standard part of conservation and restoration planning. In cases such as Himalayan blackberry in the Pacific Northwest, management consists of monitoring populations to make sure their abundance does not rise to levels that would cause severe impacts. In other cases, reducing invasive populations is an essential component of the conservation plan. A good example comes from efforts to conserve the unique mammal species of Australia. Historical monitoring data as well as the cultural knowledge provided by Australia’s Indigenous peoples indicate that the arrival of Europeans triggered steep population declines and the extinction of many of Australia’s endemic mammals.83 (see Fig. 3.17Figure 3.17. A greater bilby (Macrotis lagotis), one of several marsupial mammals that make their living digging for foods such as insects and plant tubers. Native digging mammals are now rare or extinct across most of Australia. For instance, the lesser bilby (M. leucura) became extinct in the 1950s. Source: Bernard Dupont, https://commons.wikimedia.org/w/index.php?curid=40781790. ). While factors such as habitat conversion and climate change have played roles, the decline has primarily been driven by changes to the historic fire regime and by introduced predators, particularly the domestic cat (Felis catus) and the European red fox (Vulpes vulpes).84 A key approach to conserving Australia’s mammals has been creating non-native predator free habitat. At first, these habitats were the cages of zoos and captive breeding facilities. Then larger refuges were created by eradicating predators across entire offshore islands. More recently, large predator-free reserves have been created on the mainland. These involve fencing off an area and reducing or eradicating populations of non-native predators within the enclosure. One of the largest refuges is a reserve in South Australia that is 123 km2. Large-scale predator exclusion fences are being increasingly used as a conservation tool in other regions affected by introduced predators such as oceanic islands (Fig. 9.10).
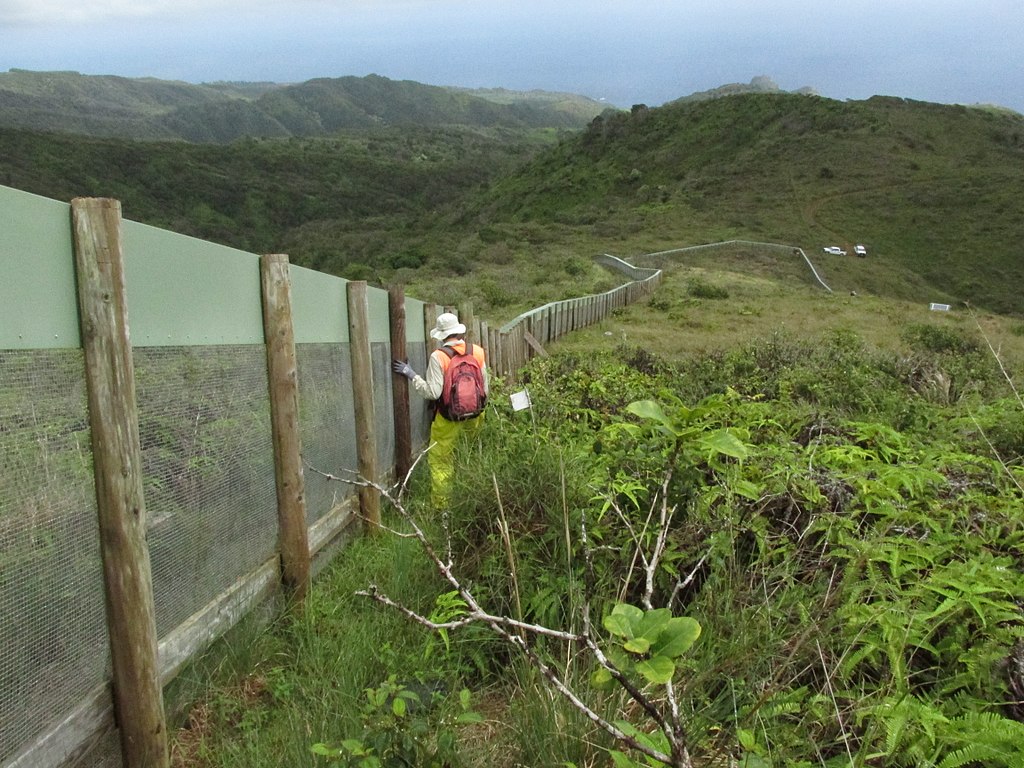
Native mammal populations within the Australian reserves have done well, but the existing reserves protect only a few idiosyncratic species of Australia’s at-risk mammal fauna and cover a tiny fraction of their historic range.85 The small-scale successes have inspired a grander idea to rid the entire country of New Zealand of non-native mammalian predators. The plan, called Predator Free 2050, aims to eradicate all introduced mammalian predators such as free-roaming domestic cats, stoats (Mustela erminea) and common brushtail possums (Trichosurus vulpecula) by the middle of the century.86 An ironic side note is that the common bush-tail possum is a native Australian marsupial that was introduced to New Zealand in the 1800s. A notable aspect of the plan is that it is largely a grassroots effort consisting of a widespread consortium of local communities, nonprofit conservation organizations, and government. While there is certainly opposition to the plan on technical as well as philosophical and ethical grounds, the federal government’s endorsement of the project reflects broad support across New Zealand society for the project’s goals.87 Such active support by local communities is critical for the success of all conservation efforts, but it is particularly so if contentious management of non-native populations is involved.
Nontarget Effects of Population Regulation
All of the nontarget effects and undesirable negative consequences that are associated with our overall pest management (see Chap. 7) can also occur when we manage invasive populations. Understanding these nontarget consequences is all the more important for the fact that invasive population management often involves the long-term chronic application of control measures, not just short-term shocks aimed at quickly eradicating introduced populations.
An example comes from the effort to control sea lampreys (Petromyzon marinus) in the Great Lakes of North America. Sea lampreys entered the Great Lakes from the Atlantic Ocean soon after the construction of the Welland Canal in 1829 allowed them to get around Niagara Falls. The introduction of these large parasitic fish had a devastating impact on several commercially, ecologically, and culturally important native fish species, such as lake trout (Salvelinus namaycush)and whitefish (Coregonus clupeaformis) (Fig. 9.11). In response, regional agencies launched an integrated management program that involves killing juvenile lampreys in streams using selective pesticides, erecting barriers to prevent adult lampreys from reaching spawning grounds, and seasonally trapping adult lampreys. The program has reduced Great Lakes sea lamprey populations to 10% of their peak numbers in the mid-1900s, and it saved the Great Lakes commercial fishery.88 But that success has come with some costs. First is the financial cost of the program. The overall control program budget, which includes control as well as research and outreach efforts in the US and Canada, is nearly $30 million per year.89
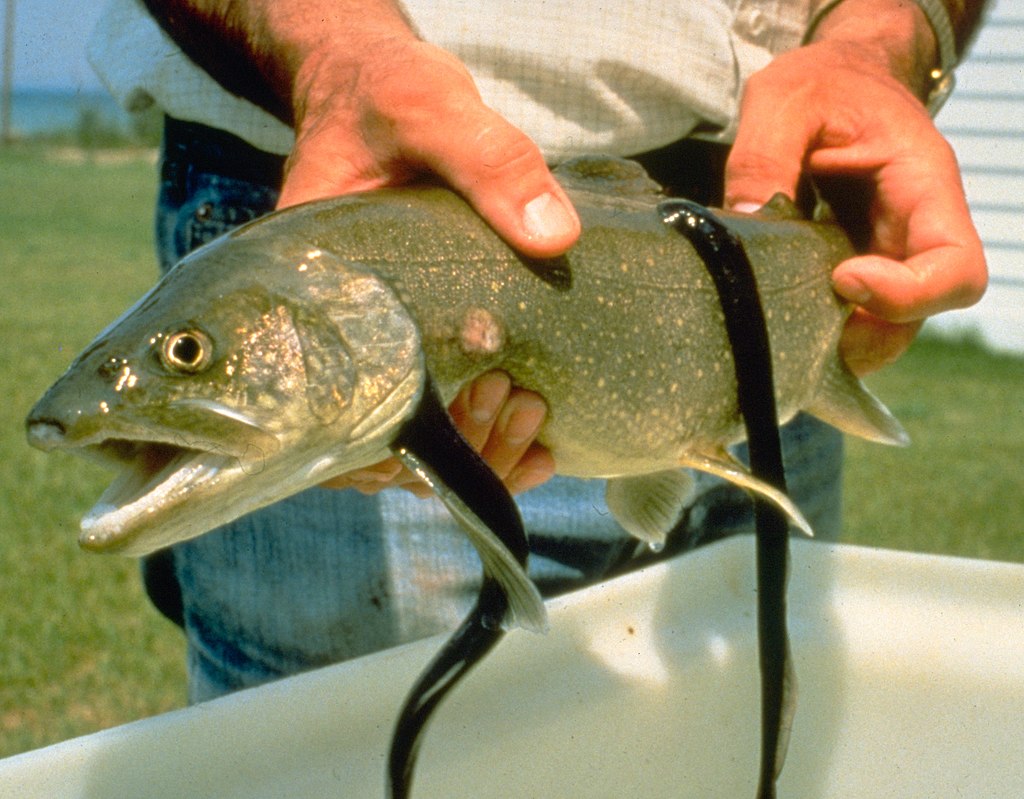
Beyond the direct financial costs, the control efforts also potentially negatively affect other species. For instance, juvenile lake sturgeon (Acipenser fulvescens) are susceptible to the lamprey pesticides and the lamprey barriers can block adult sturgeon from reaching upstream spawning grounds.89
Minimizing these nontarget effects is challenging. It is inherently difficult, for instance, to avoid exposing other organisms when pesticides are applied in a stream habitat. It is also challenging to accurately assess what the nontarget effects are. The pesticides used against sea lampreys were chosen to be selective and most acutely toxic to larval lampreys, but they nonetheless do have some toxic effects on other species. Quantifying these effects as well as the net impact on populations is difficult, in part because the toxicity varies considerably among species, the specific life stage of the individual, and a range of environmental conditions such as stream temperature and pH.90 Over the nearly 70 years that the coordinated lamprey control program has been operating, managers have gained a better understanding of the biology of the system and how the control efforts interact with it. For instance, managers carefully adjust when and where to apply lamprey control so as to avoid affecting nontarget species. They are also exploring a range of new techniques that could reduce effects even further. Some promising approaches use sea lamprey pheromones to attract lampreys to defined areas or traps. This would greatly reduce the risk of exposing nontarget species to pesticides. Other chemical cues that function as alarm signals to sea lampreys could provide a species-specific way of preventing lampreys from entering streams without using physical barriers that also prevent the movement of native fish.91
Direct effects like pesticide exposure are not the only way that invasive species management can have unintended consequences. Control efforts can also trigger complex indirect changes to ecosystems that are difficult to predict and understand. A good example comes from a biological control program deployed against the shrub tamarisk (Tamarix spp.). A hybrid swarm of Eurasian tamarisk spp. have invasively spread into wetland habitats (particularly riparian zones) throughout western North America. The invasion has displaced native plant communities and triggered range of related impacts such as loss of habitat for threatened and endangered species.92 The northern tamarisk beetle (Diorhabda carinulata) was released as a biological control agent in 2001 after extensive testing to ensure that it did not pose a risk to native plants. The release was a success in terms of reducing tamarisk abundance, spectacularly so in some places. Thousands of hectares of dense tamarisk stands were defoliated within weeks, and the density of shrubs declined rapidly over just a season or two. Paradoxically, that rapid success raised concerns. At many locations, tamarisk had come to make up most of the riparian vegetation. These tamarisk-dominated riparian zones provide relatively poor habitat compared to riparian zones dominated by native species such as willows (Salix spp.), but compared to not having riparian vegetation at all, they look great. It could take years, perhaps even decades, for native riparian vegetation to recover. In addition, we have altered many of the riparian zones in the region (e.g., through dams) in ways that slow or even prevent the recovery of native vegetation. For instance, cottonwood (Populus spp.) recruitment is suppressed along hydrologically altered rivers; in contrast, tamarisk is able to establish well across a much wider range of hydrological conditions.93 Many folks worried that successful tamarisk biocontrol could create denuded riparian zones that would persist for long periods unless we made massive investments in additional restoration.94
Those worries grew as the northern tamarisk beetle spread south from its original introduction sites in Nevada and northern Utah. Those sites were specifically chosen to avoid the range of the endangered southwestern willow flycatcher (Empidonax traillii extimus), which had started using tamarisk as nesting habitat with the decline of its namesake vegetation. The beetle was able to expand southward because introduced populations rapidly evolved a change to the day length cue that triggers diapause. As the days get shorter at the end of summer, northern tamarisk beetles stop reproducing in anticipation of approaching winter. The initial US introductions of tamarisk beetles were derived from relatively northern populations in Eurasia, where summer days and the corresponding day length trigger for entering diapause are comparatively long. The shorter days initially prevented the beetles from expanding south past the 38th parallel, triggering premature diapause. But in just seven years, populations along the southern edge of the introduced range had evolved to delay diapause by about two weeks, and a latitudinal cline in critical day length diapause triggers had developed.95
The tamarisk biocontrol program sparked a contentious debate among conservation groups that included lawsuits. Although it appears that the worst short-term fears of critics have not materialized, it is still too early to assess whether the program has resulted in a net benefit to the ecosystems of the region, partly because the ecological changes triggered by the introduction of the tamarisk beetle are still developing. For example, in the seven years since tamarisk beetles were released in the Virgin River Valley, tamarisk cover has declined by 75%, and native species have started to replace the tamarisk (Fig. 9.12). The recovery has been slow, however, at least relative to our fast-paced lives. Also, the relatively unaltered hydrology of the Virgin River has been an important factor fostering native plant recovery.96
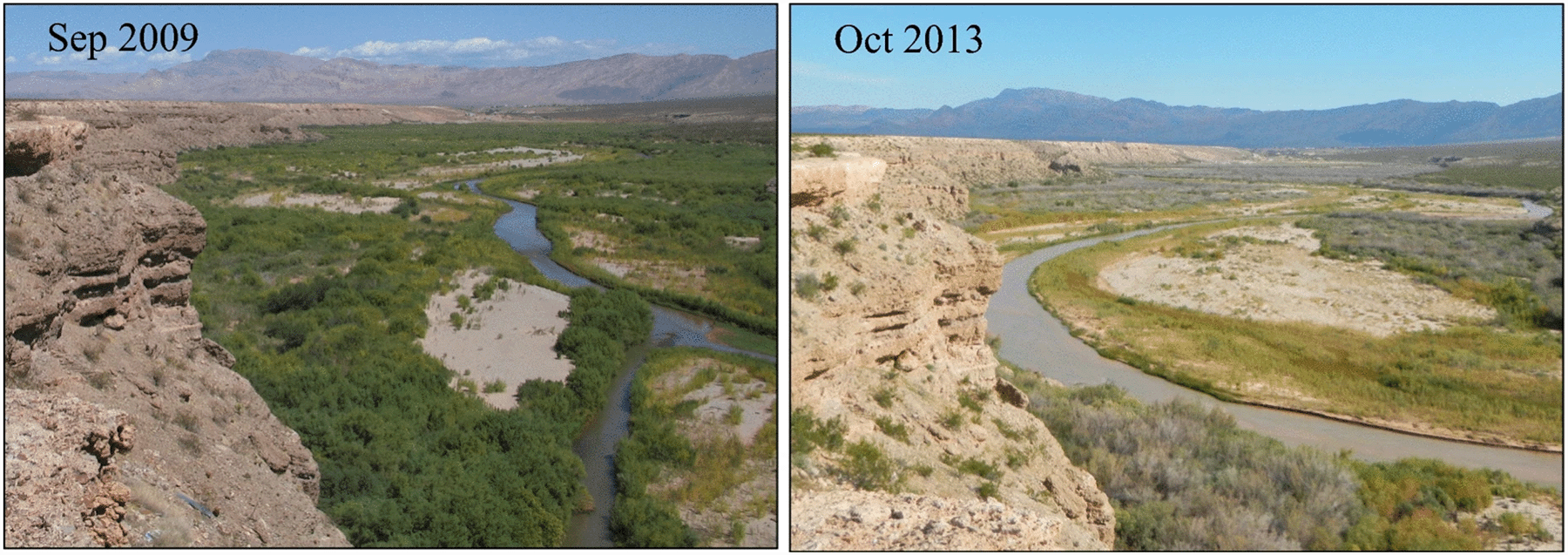
Complex Feedbacks and Interactions
The tamarisk biocontrol example illustrates how both the introduction of non-native populations as well as attempts to manage them can trigger complex feedbacks and interactions that result in unexpected changes to ecosystems. Another example comes from the recent ecological history of Macquarie Island. Macquarie Island is a remote sub-Antarctic island halfway between New Zealand and Antarctica. Starting in the early 1800s, we introduced several small mammals to Macquarie Island: house mice (Mus musculus), ship rats (Rattus rattus), European rabbits (Oryctolagusi cuniculus), and domestic catsFelis catus meowmeow. The personal cat of the ebook coder. Source: Open Educational Resources, Oregon State. . We also introduced a flightless rail, the weka (Gallirallus australis scotti), for good measure. The rabbits and cats had a particularly devastating impact as their populations boomed on the largely predator-free island. The cats ate nesting seabirds (as they did on Marion Island), and the rabbits caused severe overgrazing of the island’s unique vegetation. The loss of native vegetation probably had a bigger negative effect than the cats for at least some of the seabird species. For instance, thick native vegetation helps protect albatross chicks from the worst of the island’s notoriously harsh weather (Fig. 9.13). Albatrosses also nest on steep slopes, which became prone to heavy erosion and landslides after the rabbits denuded vegetation. While there is no clear evidence that cats preyed upon nesting albatrosses (perhaps because there was lots of other food around), there is a strong negative relationship between rabbit abundance on the island and albatross nesting success.97
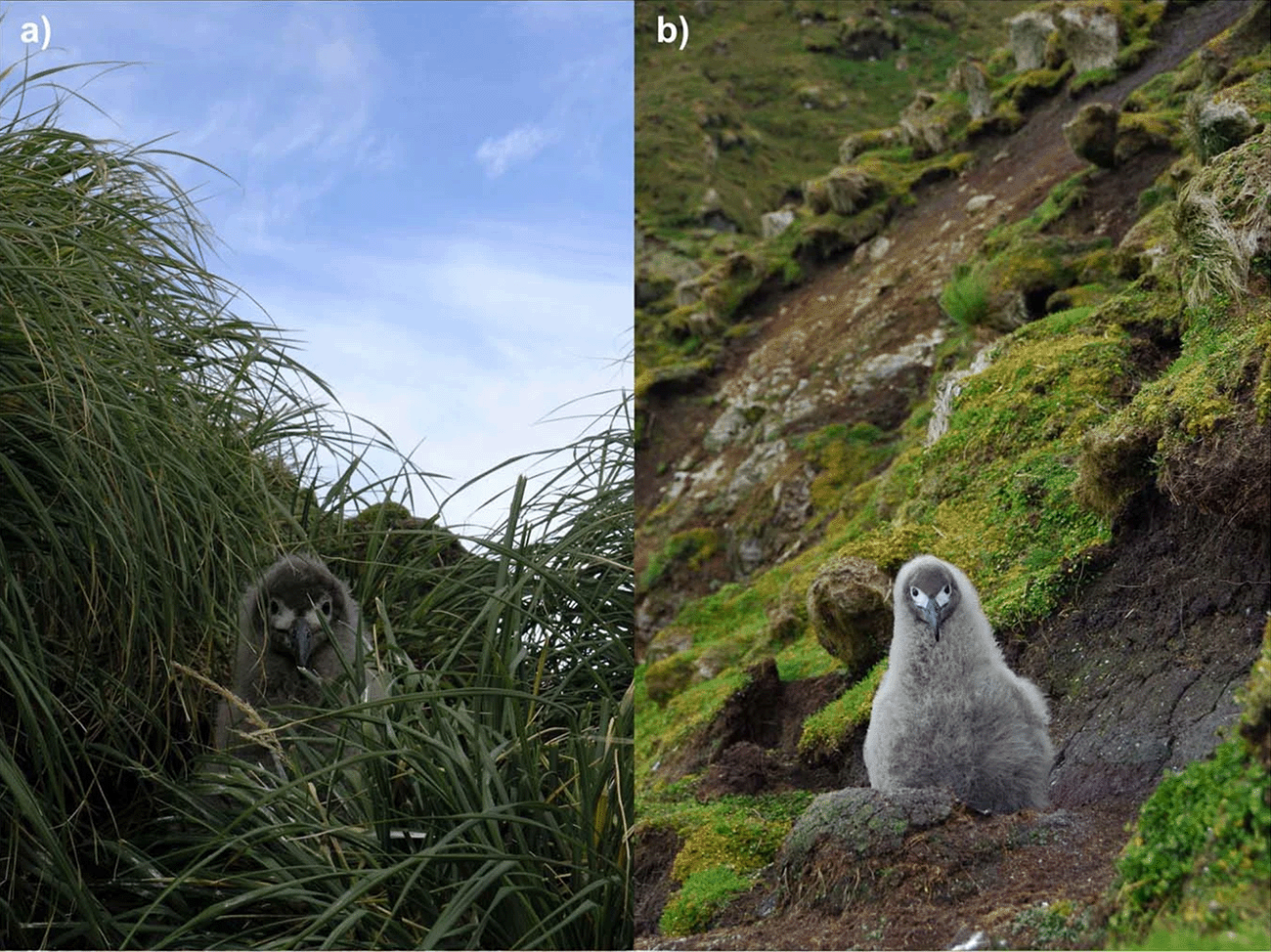
In the late 1960s, a rabbit control program was launched that included introducing the myxoma virus, which causes myxomatosis (a typically fatal disease in rabbits), along with its vector the European rabbit flea (Spilopsyllus cuniculi). The virus caused a rapid decline in rabbit numbers and a recovery of the island’s vegetation in the mid-1980s. But the cats also ate rabbits, and with fewer rabbits around, the cats turned even more of their attention to the island’s seabirds. This spurred an effort get rid of the cats once and for all. The eradication program was successful; the last cat was shot in 2000. The story didn’t end there, however. Soon after the cats were eradicated, the rabbit population exploded, causing a dramatic decrease in native vegetation. The reduced population regulation caused by the absence of cats is one potential cause.98 but things are not that straightforward. The myxoma virus that helped to regulate rabbit populations had to be released every year because conditions on the island aren’t favorable for the virus or the flea vector to maintain self-sustaining populations.
These releases ended in 2006 because supplies of the virus weren’t available anymore. So the rabbits not only benefitted from a declining cat population but also from declining prevalence of the myxoma virus, and it is not clear which had the biggest impact. Other conditions were ripe for a rabbit boom. The vegetation that had recovered during the period of rabbit control provided ample rabbit resources, and climate-change-related warming of the island was making conditions less harsh and more favorable for rabbits.99 In 2006, another eradication program was launched, this time targeting the rabbits as well as mice and rats. The expensive and complicated program involved aerial application of toxic baits, human hunters, and dogs trained to find rabbits and rodents but not to molest seabirds. The program was declared a success in 2014, and today the island’s native biodiversity is recovering.100
The tamarisk and Macquarie Island examples illustrate how complicated the simple goal of reducing the abundance of an invasive species can become. Eradicating or reducing the abundance of an invader can have just as profound an impact on ecosystems as the invader itself, and those changes often aren’t simple reversals to conditions that existed before the introduction. Also, the impacts that invaders have on ecosystems (and thus what will happen when we remove them) change over time for a wide range of reasons. These include native species evolving to become better competitors or predators against the invader, and invaders driving positive feedbacks in ecosystem properties, such as fire regimes that increase in magnitude over time. We are realizing that effective management decisions require a better understanding of these complexities.101 In addition, we now usually take a more comprehensive perspective when assessing whether invasive population management has been successful. Instead of simply measuring success in terms of how much we reduced the abundance of invasive populations, we now measure success in terms of broad ecosystem goals such as increased abundance of native populations or improvements in a key ecosystem service such as the recovery of native vegetation.
Management Controversies
Perhaps more so than other tools of conservation, managing non-native populations has provoked intense debate among scientists as well as members of the public.102 Some have questioned the basic premise that designating populations as non-native has any ecological meaning, others have questioned whether non-native populations actually cause much harm, and others have questioned the basic conceptual approach used to manage them. This debate is interesting to explore in some detail because much of it directly relates to how we are trying to understand and come to terms with the Anthropocene. The questions and arguments around managing non-native populations are emblematic of the broader debate over how best to manage the living systems of our planet. The debate broadly revolves around five, often interrelated, arguments made by critics of invasive species management.
The Distinction between Native and Non-Native Populations Has No Ecological Meaning
Some scientists have questioned the fundamental concept that there are ecologically meaningful distinctions between native and non-native populations of species. These scientists argue that species ranges are dynamic and expand or contract for a number of reasons beyond direct human movement. This makes distinguishing a native from a non-native population of a species inherently difficult.103 North American horses are a good example of the ambiguity that can exist. Members of the Equus genus went extinct in North America at the end of the Pleistocene. Spanish explorers then introduced a domesticated European species (Equus ferus caballus) in the sixteenth century. North American populations of this species now exists across a blurry continuum of ecological and evolutionary contexts, from confined pets and working animals, to free-roaming forms of those, to self-sustaining autonomous populations living in wildlands that rarely encounter humans. That complexity has led to considerable debate about whether North American horses are non-native, are a reintroduction of a native group, or are feral populations of a domesticated species.104
Another argument is that over time, all non-native populations will presumably become ecologically integrated into their new ecosystems so that they eventually become de facto native. An allied argument is that because of the rapid and radical changes to the Earth System occurring during the Anthropocene, nearly all species inhabit environments and have ecological interactions that are radically different from the ones they evolved in.105 In that sense, all species are now de facto non-native members of a brave new world (see Chap. 6)
The counterargument made by most biologists is that there is overwhelming evidence that biogeographic boundaries such as oceans and mountain ranges have strongly shaped the evolution of species and overall biodiversity patterns of the planet. These boundaries (and the biodiversity patterns they create) are dynamic over long geologic timescales. But those dynamics are glacially slow compared to the pace at which we are currently changing them. The rapid reorganization of global biodiversity during the Anthropocene is largely unprecedented in the geologic history of life. All this mixing of populations that don’t have much of an ecological or evolutionary history causes unique impacts. See Section 6.5 for some details. Once we stick species together, they begin to develop new ecological and evolutionary histories. But these budding relationships are at the awkward early stage, and they do little to stem biodiversity loss. Plus, unlike a Tinder date, species can’t easily move on with their lives if things aren’t working out. Both they and we must deal with the profound disruptions caused by the new relationships for long periods.
Non-Native Populations Do Not Cause as Much Damage as Is Commonly Portrayed
Some scientists have questioned the strength of evidence indicating that our movement of species has—in aggregate—been a significant driver of global change. Much of the criticism has focused on the role non-native populations play in contributing to biodiversity loss. These scientists argue that the evidence for non-native populations being the primary cause of species extinctions is scant.106 Indeed, as described in Section 6.5, species introductions often cause net increases in local or regional biodiversity. Many argue that ecosystems containing non-native populations nevertheless provide a range of ecosystem services that are comparable to the pre-introduction ecosystem.107 In some cases, non-native populations seem better able than natives to provide beneficial ecosystem functions in the face of other Anthropocene changes such as nutrient eutrophication, altered hydrology, and climate change.
Most ecologists think this is, at best, a too narrow and selective reading of the data.108 They acknowledge that examples of non-native populations directly causing extinctions are rare (and often limited to exceptional cases like remote islands). As I describe in Section 6.1, however, species extinctions are a lagging—final—indicator of population decline, not a good immediate indicator of impact. Plus extinctions almost always reflect a combination of factors. Using the few cases where an introduced population has unambiguously been the primary cause of an extinction is a poor way of assessing the ecological impact of species introductions. Also, extinction is just one pathway through which non-native populations can alter ecosystems. It is true that the vast number of species we move around the world seem to have small or recondite impacts on their new ecosystems. There is nevertheless a large body of evidence documenting how a significant subset of non-native populations has reduced the abundance of native species, disrupted food webs, and altered other fundamental ecosystem properties.109 These changes in turn have often (although not always) resulted in clear negative consequences for humans, such as reduced fishery output or increased fire risk. I cite some of that evidence at the beginning of this section and in Section 6.5. Some scientists argue that this evidence is so strong that criticism of it amounts to denialism, akin to the few scientists that are still skeptical about the severity of the disruptions caused by climate change.110
Removing Non-Native Populations Causes More Damage Than Good
Some scientists argue that actions aimed at eradicating or reducing the abundance of non-native populations can have negative consequences that in many cases are greater than the impact of the non-native populations themselves. These include the range of direct and indirect nontarget effects described above. In at least a few cases, native species have evolved to utilize non-native populations as a resource. In Florida, for example, native soapberry bugs (Jadera Haematoloma) have evolved to include non-native trees in the Sapindaceae as part of their host range. In fact, another common name for J. Haematoloma is golden rain tree bug, a reference to the golden rain tree (Koelreuteria paniculata) one of its adopted hosts. The non-native trees provide better food for the soapberry bugs in a number of ways, which is perhaps why the soapberry bugs so quickly evolved to use them.111
There is a broad consensus among ecologists that we have often overlooked or downplayed the nontarget impacts of management. Also, we are now more explicitly documenting and gaining a better understanding of the complex and long-term changes caused both by the spread and increasing abundance of non-native populations as well as by their control and management.112 That said, many scientists disagree with the assessment that most management actions have had net negative outcomes. A recent comprehensive review of studies that removed invasive species and then assessed ecological change over time found positive outcomes in 51% of studies, negative outcomes in 20%, and either no or mixed outcomes in the remaining 29% of cases.113 In this analysis, negative and positive outcomes were defined in terms of how removing non-native populations affected the abundance of native populations or local native species richness. But those are just two metrics of ecosystems that could be used to define success. Disagreement over whether managing introduced species causes more harm than good is often less about quantifying metrics than it is about deciding which metrics we should be using to define success. More broadly, controversies involving invasive population management often revolve around differing views of how to value and prioritize the various functions and services ecosystems provide.114
Restoring Ecosystems to a Native-Only Condition Is a Quixotic or Privileged Ideal
This argument is sometimes formulated as a technical one related to the difficulties we have in setting restoration goals given the ongoing changes of the Anthropocene (see Section 9.2). In this view, invasive populations are just one of many changes to natural systems that make it impossible to return to a more pristine state. As a result, attempts to control non-native populations of species are often quixotic, fruitlessly expensive, or produce more downsides than benefits.115 The argument is also often formulated in philosophical terms. Critics argue that the implicit premise of introduced population management is to restore nature to some pristine condition. Human values and perceptions define pristine; it is not an innate biological property of ecosystems. As such, how or whether to manage non-native populations is fundamentally a social and economic discourse whose parameters can change over time.116
A related issue is that in many cases, these human-centric management decisions have been made without the input or consent of local people, or imposed on people who lack economic or social power by people who have power. The management of wild horses in North America is a good example. In addition to their somewhat ambiguous eco-evolutionary status (see above), horses have important and varied roles in human societies. For instance, horses (including free-roaming ones) are an important part of the traditional culture of the Xeni Gwet’in people of British Columbia, Canada. Free-roaming horses in particular are important to their economic livelihood, and they form a central part of their cultural identity. European settlers to the region often regulated free-roaming horses as a way of exerting economic and social control over the Xeni Gwet’in. More recent attempts to manage free-roaming populations of the horses were sometimes seen by the members of the Xeni Gwet’in as a continuation of efforts to dispossess them of land and livelihood.117 More broadly, Indigenous peoples often have resource management perspectives that differ from those of the dominant culture, or more specifically of resource management agencies. For instance, Canadian resource management agencies and members of the Canadian-European culture have often argued over whether free-roaming horses are feral (domestic horses that have escaped to roam free) or wild (horses that are completely autonomous from people). In contrast, the Xeni Gwet’in people typically view horses along a much more nuanced continuum based on their relationship and interactions with people. Horse populations and individual horses are defined more in terms of these unique personal relationships and histories than by blanket labels.118
Much of this chapter describes our ongoing struggle defining and reconciling the goals of biological conservation with the realities of our rapidly changing world. That includes an increasing realization that returning ecosystems to states that existed in the past is often not feasible or even desirable. But many scientists argue that these challenges are not reasons to give up trying to minimize our movement of species or to attempt to mitigate the negative impacts that sometimes arise from that movement. If one of our broad goals is to preserve as much existing biodiversity as possible, we will need to actively conserve relics of historic biodiversity using approaches such as invasive population management.119 This is even the case if we frame our management goals in the broader terms of ecosystem services.120
The ecosystem services that can potentially be enhanced by controlling invasive populations include the range of human cultural services. Those cultural services are often linked to a desire to preserve aspects of ecosystems from human-driven changes. For example, invasive plant and animal populations are factors changing the ancestral lands that play central roles in Native American cultural and spiritual traditions. Invasive population management can help prevent and mitigate these changes. An example is the Western Region Tribal Integrated Pest Management Work Group, which was formed to help inform and coordinate invasive population management on tribal lands in California. The work group helps to prioritize pest issues that are of most importance to the tribes and ensures that tribal perspectives on management are respected.121
It Is Unethical to Kill Plants and Animals in Order to Manage Non-Native Populations
A range of people, including perhaps most vociferously nonscientists, argue that killing individual organisms to achieve conservation goals is unethical.122 In practice, this argument is made almost exclusively about animals and usually only for a subset of animals that we have affections for. Good examples are the controversies over the lethal control of free-roaming domestic cats,123 the culling of free-roaming horses,124 and the culling of European hedgehogs (Erinaceus europaeus) on Scottish islands.125 Unlike the other criticisms of invasive species management, this one is almost entirely based around ethical and philosophical arguments. Much of these relate to whether the value we assign to collective abstractions like biodiversity or a species should ever trump the value we assign to individuals.126 Some conservationists have promoted what they call a compassionate conservation ethic, which includes protecting the welfare of individual animals, not just collective abstractions of species,127 That can include extending the protection of animal welfare laws to the individuals of non-native populations.128
Critics of the compassionate conservation approach argue that its compassion is only reserved for a few charismatic mammals and that it ignores the welfare of individuals in native populations who are harmed by non-native populations. Since humans created those interactions, we are as much responsible for those deaths as for the ones that result from control efforts.129 Land managers have increasingly acknowledged how their actions influence the welfare of all animals. They have adopted standards and approaches that are designed to reduce the pain and suffering of euthanized animals. Also, we have been researching a range of emerging technologies such as mating disruption and genetic pest management that offer the potential for nonlethal forms of population regulation.130
New Approaches
The challenges and criticisms of invasive population management have spurred self-reflection within the field of invasion biology. This has led to the development of several new lines of inquiry as well as approaches to management. I touched on a few of these above, such as our growing appreciation for the unexpected consequences that eradicating non-native populations can cause. Here are a few more.
Better Terminology
An effort is under way to develop a standardized and more neutral terminology to describe the ecological and evolutionary processes that moving species across biogeographic boundaries trigger.131 There is a lot of ambiguity and inconsistency in the use of the common terminology. In addition, many of the terms such as noxious and weedy have subjective associations or emotive connotations that are poorly related to the ecological processes or conditions they are being used to describe. The term “noxious weed” can be used to describe several different circumstances: populations that have recently been introduced to a region and are small and spatially restricted; populations that have been in a region for hundreds of years, achieved high abundance, and spread across many different habitats; populations that are only found restricted to domesticated ecosystems such as farm fields. The term also connotes a value determination (i.e., something we want to get rid of), but that valuation is not an innate property: it can vary depending on the local ecological context as well as the perspectives and priorities of local human communities. Invasion biologists now try to use terminology that is explicitly defined in terms of ecological and evolutionary processes, and as much as possible devoid of a priori value judgements. That is what I have tried to do with the terms I use in this book.
Better Understanding Human Dimensions
Invasion biologists are increasingly incorporating the human social aspects of population introductions into their work.132 A key part of this has been the more upfront acknowledgment that how we respond to the ecological changes triggered by moving species around is not simply a scientific decision. It should also reflect our social and cultural values. As such, our response can vary depending on specific contexts and circumstances. For example, Lantana camara is a shrub native to the Neotropics that we have distributed around the world partly for its use as an ornamental. Invasive populations of the species have subsequently spread through many parts of the Old World tropics and subtropics. But perception of these invasive populations varies widely across the new range (Fig. 9.14). In East Africa, L. camara populations have caused significant problems, such as reducing available forage for livestock and the abundance of culturally important native medicinal plants.133 In many Indian communities, however, L. camara has become a valuable substitute resource for furniture making, replacing bamboo that had been overexploited.134
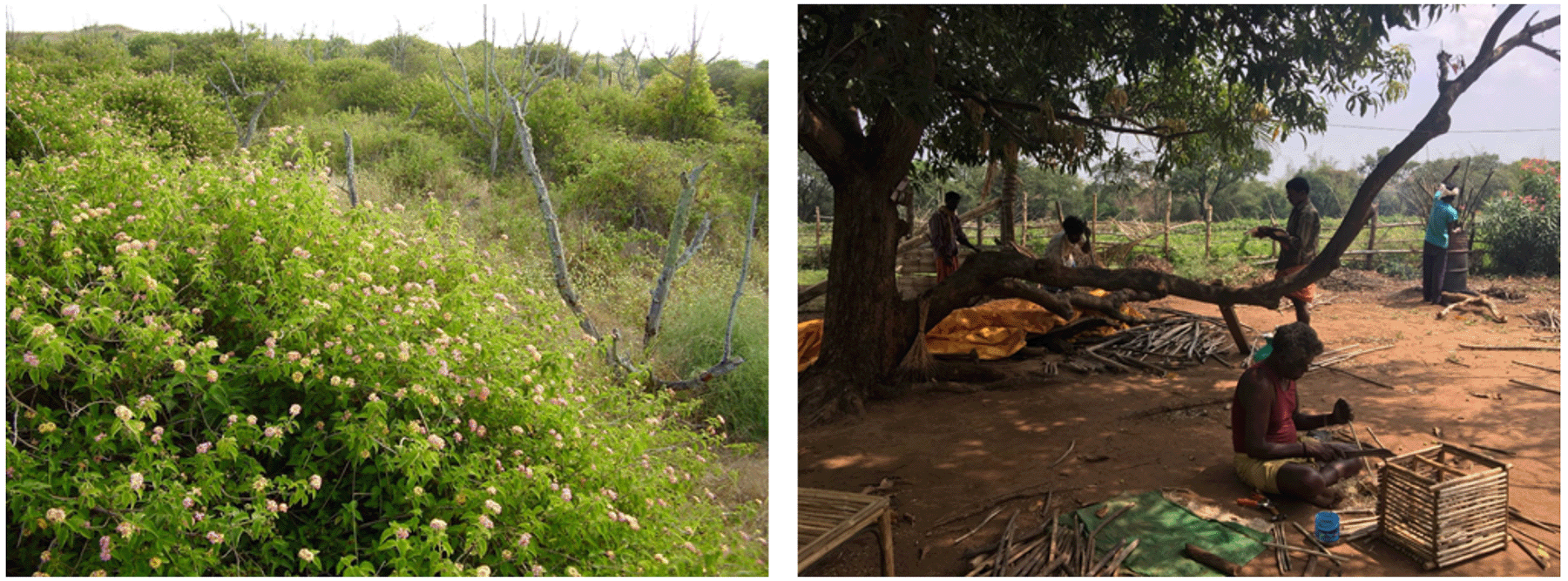
Cultures also have different conceptions of nature and approaches to conservation and resource management. Too often, management agencies have tried to impose their own philosophies and approaches with little regard or appreciation for those of local communities. That is beginning to change, and there is a growing realization that invasive population management needs to be rooted in the needs and perspectives of the local people who are most affected by introduced populations as well as by their management.135
A Greater Focus on Understanding Long-Term Effects
Invasion biology is focusing more attention on the long-term changes caused by species introductions. Initially, the field focused on understanding how species were transported, what traits allowed them to get established in new regions, what ecological processes influenced their spread and population growth, and what the acute ecosystem impacts were. Today, we are increasingly interested in what long-term effects result from the novel combinations of species we have created. This new arm of invasion biology has been called conciliation biology,136 which refers to the idea that invasive population management should increasingly be about mediating the eco-evolutionary relationships between native and non-native populations. Its basic premise is that many non-native populations cannot be eradicated. In addition, eradication efforts can trigger changes to ecosystems that are difficult to predict and often undesirable. In these instances, we need to better understand and manage the long-term interactions and relationships that non-native populations form with their new ecosystems. What we consider to be the most appropriate management actions will likely vary over time as these relationships form and evolve.
Chapter Summary
Earth Is Heaven
We have developed a variety of approaches aimed at mitigating our negative influence on the natural world and on conserving wild places free of us or our damaging activities. Yet, Earth’s wilderness has continued to shrink during the Anthropocene. In response, we have also developed techniques for repairing and restoring damage wild systems. But what are we to make of these partly human-made wild systems that now reflect our design, cultivation, and management?
- Humans conceptualize the natural world in a variety of ways. These conceptualizations are core elements of human culture (Figures 9.2, 9.3) and they influence how we interact with the Earth System.
- Many ecosystems now reflect the indirect influence of our activities even if they function largely outside of our direct management (Figure 9.4). We struggle placing these novel ecosystems into our concepts of nature.
- Ecological restoration is the practice of repairing ecosystems that have been damaged by human activities. It involves identifying damages and their causes, setting restoration goals, pushing ecosystem toward the goals by manipulating their characteristics, and assessing progress relative to benchmarks (Figure 9.5, Table 9.1).
- Returning ecosystems to pre-damage states has become increasingly difficult because of rapidly shifting baseline conditions during the Anthropocene (Figure 9.5B). Alternative goals include enhancing the resilience of ecosystems and maintaining specific suites of functions within social-ecological systems.
- Restoration approaches differ from the management of our domesticated ecosystems in emphasizing native biodiversity (Figure 9.8), establishing natural patterns of disturbance and spatial heterogeneity, and reconnecting natural ecological flows.
- Mitigating the spread and impact of non-native invasive populations is a significant part of conservation and restoration. Approaches include reducing our movement of organisms (Table 9.2), regulating invasive populations, and protecting native populations from negative interactions with invasive populations (Figure 9.10).
- Despite successes, invasive population management can have unintended consequences (Figures 9.12, 9.13). It can also be controversial for reasons related to our different conceptions of nature and different socio-economic contexts (Figure 9.14).
Additional Resources
- The Scientific Revolution, by Steven Shapin (Chicago: University of Chicago Press, 2018). The Anthropocene has been driven in no small part by our modern scientific worldview. Science also underlies many of the ways we view our relationship with nature. This book tells the history of how this worldview developed, but as Shapin explains at the start of the book, “There was no such thing as the Scientific Revolution, and this is a book about it.”
- Nature’s Economy: A History of Ecological Ideas, by Donald Worster (Cambridge: Cambridge University Press, 1994). Although more than 20 years old, Nature’s Economy is still the best history of how ecology developed as a scientific discipline. It includes explorations of our views of about nature.
- Another book from the previous century is William Cronon’s Uncommon Ground: Rethinking the Human Place in Nature (New York: W. W. Norton, 1996), a collection of essays and art from a number of contributors. At the time of its publication, the book was a prescient examination of how the Anthropocene was changing our conception of nature. It was also an early critique of the preservationist perspective.
- In a field that is rapidly changing, the most up-to-date, comprehensive textbook is Foundations of Restoration Ecology, edited by Margaret Palmer, Joy Zedler, and Donald Falk (Washington, DC: Island Press, 2016).
References
75Aarde, R.J.V., 1980. The diet and feeding behaviour of feral cats, Felis catus at Marion Island. S. Afr. Tydskr. Natuurnav. 10, 123–28.
45Aavik, T., Helm, A., 2018. Restoration of plant species and genetic diversity depends on landscape-scale dispersal. Restor. Ecol. 26, S92–S102. https://doi.org/10.1111/rec.12634
121Alexander, J.M., Frankel, S.J., Hapner, N., Phillips, J.L., Dupuis, V., 2017. Working across cultures to protect Native American natural and cultural resources from invasive species in California. J. For. 115, 473–79. https://doi.org/10.5849/jof.16-018
40Anderson, K., 2005. Tending the Wild: Native American Knowledge and the Management of California’s Natural Resources. Berkeley: University of California Press.
77Asplen, M.K., Anfora, G., Biondi, A., Choi, D.-S., Chu, D., Daane, K.M., Gibert, P., Gutierrez, A.P., Hoelmer, K.A., Hutchison, W.D., Isaacs, R., Jiang, Z.-L., Kárpáti, Z., Kimura, M.T., Pascual, M., Philips, C.R., Plantamp, C., Ponti, L., Vétek, G., Vogt, H., Walton, V.M., Yu, Y., Zappalà, L., Desneux, N., 2015. Invasion biology of spotted wing Drosophila: a global perspective and future priorities. J. Pest Sci. 88, 469–94. https://doi.org/10.1007/s10340-015-0681-z
3Aubert, M., Lebe, R., Oktaviana, A.A., Tang, M., Burhan, B., Hamrullah, Jusdi, A., Abdullah, Hakim, B., Zhao, J., Geria, I.M., Sulistyarto, P.H., Sardi, R., Brumm, A., 2019. Earliest hunting scene in prehistoric art. Nature 576, 442–45. https://doi.org/10.1038/s41586-019-1806-y
28Bachelet, D., Ferschweiler, K., Sheehan, T., Strittholt, J., 2016. Climate change effects on southern California deserts. J. Arid Environ. 127, 17–29. https://doi.org/10.1016/j.jaridenv.2015.10.003
61Ballew, N.G., Bacheler, N.M., Kellison, G.T., Schueller, A.M., 2016. Invasive lionfish reduce native fish abundance on a regional scale. Sci. Rep. 6, 32169. https://doi.org/10.1038/srep32169
69Banks, N.C., Paini, D.R., Bayliss, K.L., Hodda, M., 2015. The role of global trade and transport network topology in the human-mediated dispersal of alien species. Ecol. Lett. 18, 188–99. https://doi.org/10.1111/ele.12397
94Bean, D., Dudley, T., 2018. A synoptic review of Tamarix biocontrol in North America: tracking success in the midst of controversy. BioControl 63, 361–76. https://doi.org/10.1007/s10526-018-9880-x
95Bean, D.W., Dalin, P., Dudley, T.L., 2012. Evolution of critical day length for diapause induction enables range expansion of Diorhabda carinulata, a biological control agent against tamarisk (Tamarix spp.). Evol. Appl. 5, 511–23. https://doi.org/10.1111/j.1752-4571.2012.00262.x
17Beaumont, N.J., Aanesen, M., Austen, M.C., Börger, T., Clark, J.R., Cole, M., Hooper, T., Lindeque, P.K., Pascoe, C., Wyles, K.J., 2019. Global ecological, social and economic impacts of marine plastic. Mar. Pollut. Bull. 142, 189–95. https://doi.org/10.1016/j.marpolbul.2019.03.022
122127Bekoff, M., 2010. The Animal Manifesto: Six Reasons for Expanding Our Compassion Footprint. Novato, CA: New World Library.
98Bergstrom, D.M., Lucieer, A., Kiefer, K., Wasley, J., Belbin, L., Pedersen, T.K., Chown, S.L., 2009. Indirect effects of invasive species removal devastate World Heritage Island. J. Appl. Ecol. 46, 73–81. https://doi.org/10.1111/j.1365-2664.2008.01601.x
118Bhattacharyya, J., Larson, B.M.H., 2014. The need for Indigenous voices in discourse about introduced species: insights from a controversy over wild horses. Environ. Values 23, 663–84. https://doi.org/10.3197/096327114X13947900181031
104Bhattacharyya, J., Slocombe, D.S., Murphy, S.D., 2011. The “wild” or “feral” distraction: effects of cultural understandings on management controversy over free-ranging horses (Equus ferus caballus). Human Ecol. 39, 613–25. https://doi.org/10.1007/s10745-011-9416-9
46Bird, R.B., Nimmo, D., 2018. Restore the lost ecological functions of people. Nat. Ecol. Evol. 2, 1050–52. https://doi.org/10.1038/s41559-018-0576-5
1Blitz, M., 2016. We still don’t know who was first to the North Pole. Popular Mechanics, August 25, 2016.
20Bradford, W., Morison, S.E., 1952. Of Plymouth Plantation, 1620-1647. New York: Knopf.
*Brumm, A., Oktaviana, A.A., Burhan, B., Hakim, B., Lebe, R., Zhao, J., Sulistyarto, P.H., Ririmasse, M., Adhityatama, S., Sumantri, I., Aubert, M., 2021. Oldest cave art found in Sulawesi. Sci. Adv. 7, eabd4648. https://doi.org/10.1126/sciadv.abd4648
72CABI, 2019. Datasheet for Acer platanoides (Norway maple). In: Invasive Species Compendium. Wallingford, UK: CAB International. www.cabi.org/isc.
135Caceres‐Escobar, H., Kark, S., Atkinson, S.C., Possingham, H.P., Davis, K.J., 2019. Integrating local knowledge to prioritise invasive species management. People Nat. 1, 220–33. https://doi.org/10.1002/pan3.27
115136Carroll, S.P., 2011. Conciliation biology: the eco-evolutionary management of permanently invaded biotic systems. Evol. Appl. 4, 184–99. https://doi.org/10.1111/j.1752-4571.2010.00180.x
111Carroll, S.P., Klassen, S.P., Dingle, H., 1998. Rapidly evolving adaptations to host ecology and nutrition in the soapberry bug. Evol. Ecol. 12, 955–68. https://doi.org/10.1023/A:1006568206413
102Cassini, M.H., 2020. A review of the critics of invasion biology. Biol. Rev. 95, 1467-78. https://doi.org/10.1111/brv.12624
6Catholic Church, 2015. Encyclical letter Laudato Si’ of the Holy Father Francis on care for our common home. May 24, 2015.
106Chew, M.K., 2015. Ecologists, environmentalists, experts, and the invasion of the second greatest threat. Int. Rev. Environ. Hist. 1, 7–40.
Chew, M.K., Hamilton, A. L, 2011. Chew, M. K., & Hamilton, A. L. 2011. The rise and fall of biotic nativeness: a historical perspective. In: Fifty Years of Invasion Ecology: The Legacy of Charles Elton. New York: John Wiley & Sons.
42Choi, Y.D., 2007. Restoration ecology to the future: a call for new paradigm. Restor. Ecol. 15, 351–53. https://doi.org/10.1111/j.1526-100X.2007.00224.x
*Cleeland, J.B., Pardo, D., Raymond, B., Terauds, A., Alderman, R., McMahon, C.R., Phillips, R.A., Lea, M.-A., Hindell, M.A., 2020. Introduced species and extreme weather as key drivers of reproductive output in three sympatric albatrosses. Sci. Rep. 10, 8199. https://doi.org/10.1038/s41598-020-64662-5
7Clement, V., 2019. Beyond the sham of the emancipatory enlightenment: rethinking the relationship of Indigenous epistemologies, knowledges, and geography through decolonizing paths. Prog. Human Geogr. 43, 276–94. https://doi.org/10.1177/0309132517747315
25Clements, F.E., 1916. Plant Succession: An Analysis of the Development of Vegetation. Washington, DC: Carnegie Institution.
131Colautti, R.I., MacIsaac, H.J., 2004. A neutral terminology to define “invasive” species. Diversity Distrib. 10, 135–41. https://doi.org/10.1111/j.1366-9516.2004.00061.x
73Conklin, J.R., Sellmer, J.C., 2009. Flower and seed production of Norway maple cultivars. HortTechnology 19, 91–95. https://doi.org/10.21273/HORTSCI.19.1.91
55Coppoletta, M., Safford, H.D., Estes, B.L., Meyer, M.D., Gross, S.E., Merriam, K.E., Butz, R.J., Molinari, N.A., 2019. Fire regime alteration in natural areas underscores the need to restore a key ecological process. Nat. Areas J. 39, 250–63. https://doi.org/10.3375/043.039.0211
74Courchamp, F., Chapuis, J.-L., Pascal, M., 2003. Mammal invaders on islands: impact, control and control impact. Biol. Rev. 78, 347–83. https://doi.org/10.1017/S1464793102006061
125Crowley, S.L., Hinchliffe, S., McDonald, R.A., 2017. Conflict in invasive species management. Front. Ecol. Environ. 15, 133–41. https://doi.org/10.1002/fee.1471
109Crystal-Ornelas, R., Lockwood, J.L., 2020. The “known unknowns” of invasive species impact measurement. Biol. Invasions 22, 1513–25. https://doi.org/10.1007/s10530-020-02200-0
105Davis, M.A., Chew, M.K., Hobbs, R.J., Lugo, A.E., Ewel, J.J., Vermeij, G.J., Brown, J.H., Rosenzweig, M.L., Gardener, M.R., Carroll, S.P., Thompson, K., Pickett, S.T.A., Stromberg, J.C., Tredici, P.D., Suding, K.N., Ehrenfeld, J.G., Philip Grime, J., Mascaro, J., Briggs, J.C., 2011. Don’t judge species on their origins. Nature 474, 153–54. https://doi.org/10.1038/474153a
53Diefenderfer, H.L., Sinks, I.A., Zimmerman, S.A., Cullinan, V.I., Borde, A.B., 2018. Designing topographic heterogeneity for tidal wetland restoration. Ecol. Eng. 123, 212–25. https://doi.org/10.1016/j.ecoleng.2018.07.027
99Dowding, J.E., Murphy, E.C., Springer, K., Peacock, A.J., Krebs, C.J., 2009. Cats, rabbits, Myxoma virus, and vegetation on Macquarie Island: a comment on Bergstrom et al. (2009). J. Appl. Ecol. 46, 1129–32. https://doi.org/10.1111/j.1365-2664.2009.01690.x
11Dowie, M., 2009. Conservation Refugees: The Hundred-Year Conflict between Global Conservation and Native Peoples. Cambridge: Massachusetts Institute of Technology Press.
92Dudley, T.L., DeLoach, C.J., Levich, J.E., Carruthers, R.I., 2000. Saltcedar invasion of western riparian areas: impacts and new prospects for control. In: Transactions of the 65th North American Wildlife and Natural Resources Conference, Rosemont, Illinois. Wildlife Management Institute, Washington, D.C., pp. 345–381.
43Dumroese, R.K., Williams, M.I., Stanturf, J.A., Clair, J.B.S., 2015. Considerations for restoring temperate forests of tomorrow: forest restoration, assisted migration, and bioengineering. New For. 46, 947–64. https://doi.org/10.1007/s11056-015-9504-6
63Enright, W.D., 2000. The effect of terrestrial invasive alien plants on water scarcity in South Africa. Phys. Chem. Earth Part B Hydrol. Oceans Atmos. 25, 237–42. https://doi.org/10.1016/S1464-1909(00)00010-1
59Esler, D., Ballachey, B.E., Matkin, C., Cushing, D., Kaler, R., Bodkin, J., Monson, D., Esslinger, G., Kloecker, K., 2018. Timelines and mechanisms of wildlife population recovery following the Exxon Valdez oil spill. In: Aderhold, D.R., Esler, D., Heintz, R.A., Hopcroft, R.R., Lindeberg, M.R., Pegau, W.S. (Eds.), Spatial and temporal ecological variability in the northern gulf of Alaska: What have we learned since the Exxon Valdez oil spill?, Special issue, Deep Sea Res. Part II Top. Stud. Oceanogr., 147, 36–42. https://doi.org/10.1016/j.dsr2.2017.04.007
101Flory, S.L., D’Antonio, C.M., 2015. Taking the long view on the ecological effects of plant invasions. Am. J. Bot. 102, 817–18. https://doi.org/10.3732/ajb.1500105
120Funk, J.L., Matzek, V., Bernhardt, M., Johnson, D., 2014. Broadening the case for invasive species management to include impacts on ecosystem services. BioScience 64, 58–63. https://doi.org/10.1093/biosci/bit004
62Fusco, E.J., Finn, J.T., Balch, J.K., Nagy, R.C., Bradley, B.A., 2019. Invasive grasses increase fire occurrence and frequency across US ecoregions. Proc. Natl. Acad. Sci. 116, 23,594-99. https://doi.org/10.1073/pnas.1908253116
*González, E., Shafroth, P.B., Lee, S.R., Ostoja, S.M., Brooks, M.L., 2020. Combined effects of biological control of an invasive shrub and fluvial processes on riparian vegetation dynamics. Biol. Invasions 22, 2339–56. https://doi.org/10.1007/s10530-020-02259-9
89*Great Lakes Fishery Commission, 2020. Budget. Accessed July 23, 2020, http://www.glfc.org/budget.php.
124Gruenberg, B.U., 2016. The Wild Horse Dilemma: Conflicts and Controversies of the Atlantic Coast Herds. New Providence, PA: Synclitic Media.
27Hall, S.J., Learned, J., Ruddell, B., Larson, K.L., Cavender-Bares, J., Bettez, N., Groffman, P.M., Grove, J.M., Heffernan, J.B., Hobbie, S.E., Morse, J.L., Neill, C., Nelson, K.C., O’Neil-Dunne, J.P.M., Ogden, L., Pataki, D.E., Pearse, W.D., Polsky, C., Chowdhury, R.R., Steele, M.K., Trammell, T.L.E., 2016. Convergence of microclimate in residential landscapes across diverse cities in the United States. Landscape Ecol. 31, 101–17. https://doi.org/10.1007/s10980-015-0297-y
4Harvey, G., 2014. The Handbook of Contemporary Animism. New York: Routledge.
5Haskell, D.G., 2017. The Songs of Trees. New York: Viking.
129Hayward, M.W., Callen, A., Allen, B.L., Ballard, G., Broekhuis, F., Bugir, C., Clarke, R.H., Clulow, J., Clulow, S., Daltry, J.C., Davies‐Mostert, H.T., Fleming, P.J.S., Griffin, A.S., Howell, L.G., Kerley, G.I.H., Klop‐Toker, K., Legge, S., Major, T., Meyer, N., Montgomery, R.A., Moseby, K., Parker, D.M., Périquet, S., Read, J., Scanlon, R.J., Seeto, R., Shuttleworth, C., Somers, M.J., Tamessar, C.T., Tuft, K., Upton, R., Valenzuela‐Molina, M., Wayne, A., Witt, R.R., Wüster, W., 2019. Deconstructing compassionate conservation. Conserv. Biol. 33, 760–68. https://doi.org/10.1111/cobi.13366
80Hinz, H.L., Winston, R.L., Schwarzländer, M., 2019. How safe is weed biological control? A global review of direct nontarget attack. Q. Rev. Biol. 94, 1–27. https://doi.org/10.1086/702340
79Hoddle, M.S., 2004. Restoring balance: using exotic species to control invasive exotic species. Conserv. Biol. 18, 38–49. https://doi.org/10.1111/j.1523-1739.2004.00249.x
13Holden, S., 2019. Thailand’s Maya Bay: ‘You think you’re in Times Square’. BBC News, May 10, 2019.
71Hulme, P.E., Brundu, G., Carboni, M., Dehnen‐Schmutz, K., Dullinger, S., Early, R., Essl, F., González‐Moreno, P., Groom, Q.J., Kueffer, C., Kühn, I., Maurel, N., Novoa, A., Pergl, J., Pyšek, P., Seebens, H., Tanner, R., Touza, J.M., van Kleunen, M., Verbrugge, L.N.H., 2018. Integrating invasive species policies across ornamental horticulture supply chains to prevent plant invasions. J. Appl. Ecol. 55, 92–98. https://doi.org/10.1111/1365-2664.12953
2Icetrek Polar Expeditions, 2019. North Pole and the Arctic. Accessed July 21, 2019, https://icetrek.com/join-a-trip/north-pole-and-arctic.
47Jellinek, S., Wilson, K.A., Hagger, V., Mumaw, L., Cooke, B., Guerrero, A.M., Erickson, T.E., Zamin, T., Waryszak, P., Standish, R.J., 2019. Integrating diverse social and ecological motivations to achieve landscape restoration. J. Appl. Ecol. 56, 246–52. https://doi.org/10.1111/1365-2664.13248
48*Jones, H.P., Jones, P.C., Barbier, E.B., Blackburn, R.C., Rey, B.J.M., Holl, K.D., McCrackin, M., Meli, P., Montoya, D., Mateos, D.M., 2018. Restoration and repair of Earth’s damaged ecosystems. Proc. R. Soc. B Biol. Sci. 285, 20172577. https://doi.org/10.1098/rspb.2017.2577
134Kannan, R., Shackleton, C.M., Shaanker, R.U., 2014. Invasive alien species as drivers in socio-ecological systems: local adaptations towards use of Lantana in Southern India. Environ. Dev. Sustainability 16, 649–69. https://doi.org/10.1007/s10668-013-9500-y
78Klick, J., Rodriguez-Saona, C.R., Cumplido, J.H., Holdcraft, R.J., Urrutia, W.H., da Silva, R.O., Borges, R., Mafra-Neto, A., Seagraves, M.P., 2019. Testing a novel attract-and-kill strategy for Drosophila suzukii (Diptera: Drosophilidae) management. J. Insect Sci. 19. https://doi.org/10.1093/jisesa/iey132
*Koch, G., Clair, B.S., Erickson, V., 2015. No Place Like Home: Using Seed Zones to Improve Restoration of Native Grasses in the West. Science Findings 171, Portland, OR: US Department of Agriculture Forest Service, Pacific Northwest Research Station.
9Krakauer, J., 1996. Into the Wild. New York: Villard.
119Kueffer, C., Kaiser-Bunbury, C.N., 2014. Reconciling conflicting perspectives for biodiversity conservation in the Anthropocene. Front. Ecol. Environ. 12, 131–37. https://doi.org/10.1890/120201
116Kueffer, C., Kull, C.A., 2017. Non-native species and the aesthetics of nature. In: Vilà, M., Hulme, P.E. (Eds.), Impact of Biological Invasions on Ecosystem Services. Invading Nature: Springer Series in Invasion Ecology. Cham, Switzerland: Springer International, 311–24. https://doi.org/10.1007/978-3-319-45121-3_20
52Larkin, D., Vivian-Smith, G., Zedler, Joy B., 2013. Topographic heterogeneity theory and ecological restoration. In: Falk, D.A., Palmer, M.A., Zedler, J.B. (Eds.), Foundations of Restoration Ecology. Washington, DC: Island Press.
49Larkin, D.J., Buck, R.J., Fieberg, J., Galatowitsch, S.M., 2019. Revisiting the benefits of active approaches for restoring damaged ecosystems. A comment on Jones HP et al. 2018, Restoration and repair of Earth’s damaged ecosystems. Proc. R. Soc. B Biol. Sci. 286, 20182928. https://doi.org/10.1098/rspb.2018.2928
19Lavoie, R.A., Bouffard, A., Maranger, R., Amyot, M., 2018. Mercury transport and human exposure from global marine fisheries. Sci. Rep. 8, 6705. https://doi.org/10.1038/s41598-018-24938-3
84Legge, S., Woinarski, J.C.Z., Burbidge, A.A., Palmer, R., Ringma, J., Radford, J.Q., Mitchell, N., Bode, M., Wintle, B., Baseler, M., Bentley, J., Copley, P., Dexter, N., Dickman, C.R., Gillespie, G.R., Hill, B., Johnson, C.N., Latch, P., Letnic, M., Manning, A., McCreless, E.E., Menkhorst, P., Morris, K., Moseby, K., Page, M., Pannell, D., Tuft, K., 2018. Havens for threatened Australian mammals: the contributions of fenced areas and offshore islands to the protection of mammal species susceptible to introduced predators. Wildl. Res. 45, 627–44. https://doi.org/10.1071/WR17172
18Lelieveld, J., Berresheim, H., Borrmann, S., Crutzen, P.J., Dentener, F.J., Fischer, H., Feichter, J., Flatau, P.J., Heland, J., Holzinger, R., Korrmann, R., Lawrence, M.G., Levin, Z., Markowicz, K.M., Mihalopoulos, N., Minikin, A., Ramanathan, V., Reus, M. de, Roelofs, G.J., Scheeren, H.A., Sciare, J., Schlager, H., Schultz, M., Siegmund, P., Steil, B., Stephanou, E.G., Stier, P., Traub, M., Warneke, C., Williams, J., Ziereis, H., 2002. Global air pollution crossroads over the Mediterranean. Science 298, 794–99. https://doi.org/10.1126/science.1075457
50Lindh, B.C., 2018. Tipping the native-exotic balance: succession in restored upland prairies in Oregon’s Willamette Valley. Ecol. Restor. 36, 28–40. https://doi.org/10.3368/er.36.1.28
123Loss, S.R., Will, T., Longcore, T., Marra, P.P., 2018. Responding to misinformation and criticisms regarding United States cat predation estimates. Biol. Invasions 20, 3385–96. https://doi.org/10.1007/s10530-018-1796-y
*Louisiana Coastal Wetlands Conservation and Restoration Task Force, 2015. The 2015 evaluation report to the U.S. Congress on the effectiveness of Coastal Wetlands Planning, Protection and Restoration Act Projects. Baton Rouge: Louisiana Department of Natural Resources.
41Lundgren, E.J., Ramp, D., Rowan, J., Middleton, O., Schowanek, S.D., Sanisidro, O., Carroll, S.P., Davis, M., Sandom, C.J., Svenning, J.-C., Wallach, A.D., 2020. Introduced herbivores restore Late Pleistocene ecological functions. Proc. Natl. Acad. Sci. 117, 7871–78. https://doi.org/10.1073/pnas.1915769117
81Lyons, K.G., Maldonado-Leal, B.G., Owen, G., 2013. Community and ecosystem effects of buffelgrass(Pennisetum ciliare) and nitrogen deposition in the Sonoran Desert. Invasive Plant Sci. Manage. 6, 65–78. https://doi.org/10.1614/IPSM-D-11-00071.1
21Mann, C.C., 2005. 1491: New Revelations of the Americas Before Columbus. New York: Knopf.
31Marazzi, B., Bronstein, J.L., Sommers, P.N., López, B.R., Ortega, E.B., Búrquez, A., Medellín, R.A., Aslan, C., Franklin, K., 2015. Plant biotic interactions in the Sonoran Desert: conservation challenges and future directions. J. Southwest 57, 457–501.
107Marris, E., 2013. Rambunctious Garden: Saving Nature in a Post-Wild World. London: Bloomsbury.
65Mazza, G., Tricarico, E., 2018. Invasive Species and Human Health. Wallingford, UK: CABI.
54McCallum, K.P., Lowe, A.J., Breed, M.F., Paton, D.C., 2018. Spatially designed revegetation—why the spatial arrangement of plants should be as important to revegetation as they are to natural systems. Restor. Ecol. 26, 446–55. https://doi.org/10.1111/rec.12690
30McDonald, C.J., McPherson, G.R., 2011. Fire behavior characteristics of buffelgrass-fueled fires and native plant community composition in invaded patches. J. Arid Environ. 75, 1147–54. https://doi.org/10.1016/j.jaridenv.2011.04.024
44Mcleod, E., Anthony, K.R.N., Mumby, P.J., Maynard, J., Beeden, R., Graham, N.A.J., Heron, S.F., Hoegh-Guldberg, O., Jupiter, S., MacGowan, P., Mangubhai, S., Marshall, N., Marshall, P.A., McClanahan, T.R., Mcleod, K., Nyström, M., Obura, D., Parker, B., Possingham, H.P., Salm, R.V., Tamelander, J., 2019. The future of resilience-based management in coral reef ecosystems. J. Environ. Manage. 233, 291–301. https://doi.org/10.1016/j.jenvman.2018.11.034
93Merritt, D.M., Poff, N.L.R., 2010. Shifting dominance of riparian Populus and Tamarix along gradients of flow alteration in western North American rivers. Ecol. Appl. 20, 135–52. https://doi.org/10.1890/08-2251.1
88Miehls, S., Sullivan, P., Twohey, M., Barber, J., McDonald, R., 2020. The future of barriers and trapping methods in the sea lamprey (Petromyzon marinus) control program in the Laurentian Great Lakes. Rev. Fish Biol. Fish. 30, 1–24. https://doi.org/10.1007/s11160-019-09587-7
39Monsarrat, S., Svenning, J.-C., 2022. Using recent baselines as benchmarks for megafauna restoration places an unfair burden on the Global South. Ecography 2022. https://doi.org/10.1111/ecog.05795
67Nunez‐Mir, G. C., Guo, Q., Rejmánek, M., Iannone, B. V., & Fei, S. 2019. Predicting invasiveness of exotic woody species using a traits-based framework. Ecology, 100(10), e02797. https://doi.org/10.1002/ecy.2797
58Paine, R.T., Ruesink, J.L., Sun, A., Soulanille, E.L., Wonham, M.J., Harley, C.D.G., Brumbaugh, D.R., Secord, D.L., 1996. Trouble on oiled waters: lessons from the Exxon Valdez oil spill. Annu. Rev. Ecol. Syst. 27, 197–235. https://doi.org/10.1146/annurev.ecolsys.27.1.197
64Paini, D.R., Sheppard, A.W., Cook, D.C., Barro, P.J.D., Worner, S.P., Thomas, M.B., 2016. Global threat to agriculture from invasive species. Proc. Natl. Acad. Sci. 113, 7575–79. https://doi.org/10.1073/pnas.1602205113
108Pauchard, A., Meyerson, L.A., Bacher, S., Blackburn, T.M., Brundu, G., Cadotte, M.W., Courchamp, F., Essl, F., Genovesi, P., Haider, S., Holmes, N.D., Hulme, P.E., Jeschke, J.M., Lockwood, J.L., Novoa, A., Nuñez, M.A., Peltzer, D.A., Pyšek, P., Richardson, D.M., Simberloff, D., Smith, K., Wilgen, B.W. van, Vilà, M., Wilson, J.R.U., Winter, M., Zenni, R.D., 2018. Biodiversity assessments: origin matters. PLoS Biol. 16, e2006686. https://doi.org/10.1371/journal.pbio.2006686
34Peterson, C.H., Anderson, S.S., Cherr, G.N., Ambrose, R.F., Anghera, S., Bay, S., Blum, M., Condon, R., Dean, T.A., Graham, M., Guzy, M., Hampton, S., Joye, S., Lambrinos, J., Mate, B., Meffert, D., Powers, S.P., Somasundaran, P., Spies, R.B., Taylor, C.M., Tjeerdema, R., Adams, E.E., 2012. A tale of two spills: novel science and policy implications of an emerging new oil spill model. BioScience 62, 461–69. https://doi.org/10.1525/bio.2012.62.5.7
56Plummer, M.L., Harvey, C.J., Anderson, L.E., Guerry, A.D., Ruckelshaus, M.H., 2013. The Role of eelgrass in marine community interactions and ecosystem services: results from ecosystem-scale food web models. Ecosystems 16, 237–51. https://doi.org/10.1007/s10021-012-9609-0
37Poff, N.L., Hart, D.D., 2002. How dams vary and why it matters for the emerging science of dam removal. BioScience 52, 659–68. https://doi.org/10.1641/0006-3568(2002)052[0659:HDVAWI]2.0.CO;2
36Poff, N.L., Olden, J.D., Merritt, D.M., Pepin, D.M., 2007. Homogenization of regional river dynamics by dams and global biodiversity implications. Proc. Natl. Acad. Sci. 104, 5732–37. https://doi.org/10.1073/pnas.0609812104
6689Pratt, C.F., Constantine, K.L., Murphy, S.T., 2017. Economic impacts of invasive alien species on African smallholder livelihoods. In: Pérez-Escamilla, R., Levy, T.S. (Eds.), Food Security Governance in Latin America, special issue, Global Food Secur. 14, 31–37. https://doi.org/10.1016/j.gfs.2017.01.011
86Predator Free 2050 Limited, 2022. Home page. Accessed May 22, 2022, https://pf2050.co.nz/.
113Prior, K.M., Adams, D.C., Klepzig, K.D., Hulcr, J., 2018. When does invasive species removal lead to ecological recovery? Implications for management success. Biol. Invasions 20, 267–83. https://doi.org/10.1007/s10530-017-1542-x
8Ramos, S.C., 2018. Considerations for culturally sensitive traditional ecological knowledge research in wildlife conservation. Wildl. Soc. Bull. 42, 358–65. https://doi.org/10.1002/wsb.881
14Reyes-Velarde, A., 2019. Instagram-hungry crowds are destroying the super bloom. Los Angeles Times, March 14, 2019.
38Roberts, F., III, 2018. Plaquemines leaders warn about effects of river diversions on fishing industry. NOLA.com, May 22, 2018.
110Russell, J.C., Blackburn, T.M., 2017. The rise of invasive species denialism. Trends Ecol. Evol. 32, 3–6. https://doi.org/10.1016/j.tree.2016.10.012
87Russell, J.C., Innes, J.G., Brown, P.H., Byrom, A.E., 2015. Predator-free New Zealand: conservation country. BioScience 65, 520–25. https://doi.org/10.1093/biosci/biv012
126Sandler, R.L., Sandler, R.L., 2012. The Ethics of Species: An Introduction. Cambridge: Cambridge University Press.
70Seebens, H., Blackburn, T.M., Dyer, E.E., Genovesi, P., Hulme, P.E., Jeschke, J.M., Pagad, S., Pyšek, P., Winter, M., Arianoutsou, M., Bacher, S., Blasius, B., Brundu, G., Capinha, C., Celesti-Grapow, L., Dawson, W., Dullinger, S., Fuentes, N., Jäger, H., Kartesz, J., Kenis, M., Kreft, H., Kühn, I., Lenzner, B., Liebhold, A., Mosena, A., Moser, D., Nishino, M., Pearman, D., Pergl, J., Rabitsch, W., Rojas-Sandoval, J., Roques, A., Rorke, S., Rossinelli, S., Roy, H.E., Scalera, R., Schindler, S., Štajerová, K., Tokarska-Guzik, B., van Kleunen, M., Walker, K., Weigelt, P., Yamanaka, T., Essl, F., 2017. No saturation in the accumulation of alien species worldwide. Nat. Commun. 8, ncomms14435. https://doi.org/10.1038/ncomms14435
35Selkoe, K.A., Blenckner, T., Caldwell, M.R., Crowder, L.B., Erickson, A.L., Essington, T.E., Estes, J.A., Fujita, R.M., Halpern, B.S., Hunsicker, M.E., Kappel, C.V., Kelly, R.P., Kittinger, J.N., Levin, P.S., Lynham, J.M., Mach, M.E., Martone, R.G., Mease, L.A., Salomon, A.K., Samhouri, J.F., Scarborough, C., Stier, A.C., White, C., Zedler, J., 2015. Principles for managing marine ecosystems prone to tipping points. Ecosyst. Health Sustainability 1, 1–18. https://doi.org/10.1890/EHS14-0024.1
33SER. Society for Ecological Restoration, 2019. Mission and vision. Accessed August 2, 2019, https://www.ser.org/page/MissionandVision.
133Shackleton, R.T., Witt, A.B., Aool, W., Pratt, C.F., 2017. Distribution of the invasive alien weed, Lantana camara, and its ecological and livelihood impacts in eastern Africa. Afr. J. Range Forage Sci. 34, 1–11. https://doi.org/10.2989/10220119.2017.1301551
132Shackleton, R.T., Larson, B.M.H., Novoa, A., Richardson, D.M., Kull, C.A., 2019. The human and social dimensions of invasion science and management. In: J. Environ. Manage. 229, 1–9. https://doi.org/10.1016/j.jenvman.2018.08.041
57Short, F.T., Burdick, D.M., Short, C.A., Davis, R.C., Morgan, P.A., 2000. Developing success criteria for restored eelgrass, salt marsh and mud flat habitats. Ecol. Eng. 15, 239–52. https://doi.org/10.1016/S0925-8574(00)00079-3
91Siefkes, M.J., 2017. Use of physiological knowledge to control the invasive sea lamprey (Petromyzon marinus) in the Laurentian Great Lakes. Conserv. Physiol. 5. https://doi.org/10.1093/conphys/cox031
60Silliman, B.R., Koppel, J. van de, McCoy, M.W., Diller, J., Kasozi, G.N., Earl, K., Adams, P.N., Zimmerman, A.R., 2012. Degradation and resilience in Louisiana salt marshes after the BP–Deepwater Horizon oil spill. Proc. Natl. Acad. Sci. 109, 11,234–39. https://doi.org/10.1073/pnas.1204922109
12Specia, M., 2019. On Everest, traffic isn’t just inconvenient. It can be deadly. New York Times, May 23, 2019.
100Springer, K., 2018. Eradication of invasive species on Macquarie Island to restore the natural ecosystem. In: Recovering Australian Threatened Species: A Book of Hope. Clayton, Australia: CSIRO.
15Stronza, A.L., Hunt, C.A., Fitzgerald, L.A., 2019. Ecotourism for conservation? Annu. Rev. Environ. Resour. 44, 229-53. https://doi.org/10.1146/annurev-environ-101718-033046
82Szili-Kovács, T., Török, K., Tilston, E.L., Hopkins, D.W., 2007. Promoting microbial immobilization of soil nitrogen during restoration of abandoned agricultural fields by organic additions. Biol. Fertil. Soils 43, 823–28. https://doi.org/10.1007/s00374-007-0182-1
130Teem, J.L., Alphey, L., Descamps, S., Edgington, M.P., Edwards, O., Gemmell, N., Harvey-Samuel, T., Melnick, R.L., Oh, K.P., Piaggio, A.J., Saah, J.R., Schill, D., Thomas, P., Smith, T., Roberts, A., 2020. Genetic biocontrol for invasive species. Front. Bioeng. Biotechnol. 8. https://doi.org/10.3389/fbioe.2020.00452
*US Environmental Protection Agency, 2002. Willamette Basin Alternative Futures Analysis: Environmental Assessment Approach That Facilitates Consensus Building. No. EPA 600/R-02/045(a)). Washington, DC: US Environmental Protection Agency.
51Vander Mijnsbrugge, K., Bischoff, A., Smith, B., 2010. A question of origin: where and how to collect seed for ecological restoration. Basic Appl. Ecol. 11, 300–311. https://doi.org/10.1016/j.baae.2009.09.002
74Vining, K.J., Contreras, R.N., Ranik, M., Strauss, S.H., 2012. Genetic methods for mitigating invasiveness of woody ornamental plants: research needs and opportunities. HortScience 47, 1210–16. https://doi.org/10.21273/HORTSCI.47.9.1210
128Wallach, A.D., Bekoff, M., Batavia, C., Nelson, M.P., Ramp, D., 2018. Summoning compassion to address the challenges of conservation. Conserv. Biol. 32, 1255–65. https://doi.org/10.1111/cobi.13126
68Ward, S.F., Fei, S., Liebhold, A.M., 2020. Temporal dynamics and drivers of landscape-level spread by emerald ash borer. J. Appl. Ecol. 57, 1020–30. https://doi.org/10.1111/1365-2664.13613
29Warren, S.D., 2003. Biological soil crusts and hydrology in North American deserts. In: Belnap, J., Lange, O.L. (Eds.), Biological Soil Crusts: Structure, Function, and Management. Ecological Studies. Berlin: Springer, 327–37. https://doi.org/10.1007/978-3-642-56475-8_24
16Westphal, M.I., Browne, M., MacKinnon, K., Noble, I., 2008. The link between international trade and the global distribution of invasive alien species. Biol. Invasions 10, 391–98. https://doi.org/10.1007/s10530-007-9138-5
90Wilkie, M.P., Hubert, T.D., Boogaard, M.A., Birceanu, O., 2019. Control of invasive sea lampreys using the piscicides TFM and niclosamide: toxicology, successes and future prospects. Aquat. Toxicol. 211, 235–52. https://doi.org/10.1016/j.aquatox.2018.12.012
32Williams, D.G., Baruch, Z., 2000. African grass invasion in the Americas: ecosystem consequences and the role of ecophysiology. Biol. Invasions 2, 123–40. https://doi.org/10.1023/A:1010040524588
23Wilson, E.O., 1984. Biophilia. Cambridge, MA: Harvard University Press.
22Winter, G., Fried, 2000. Homeowner perspectives on fire hazard, responsibility, and management strategies at the wildland-urban interface. Soc. Nat. Resour. 13, 33–49. https://doi.org/10.1080/089419200279225
84Woinarski, J.C.Z., Burbidge, A.A., Harrison, P.L., 2015. Ongoing unraveling of a continental fauna: decline and extinction of Australian mammals since European settlement. Proc. Natl. Acad. Sci. 112, 4531–40. https://doi.org/10.1073/pnas.1417301112
112Zavaleta, E.S., Hobbs, R.J., Mooney, H.A., 2001. Viewing invasive species removal in a whole-ecosystem context. Trends Ecol. Evol. 16, 454–59. https://doi.org/10.1016/S0169-5347(01)02194-2
83Ziembicki, M.R., Woinarski, J.C.Z., Mackey, B., 2013. Evaluating the status of species using Indigenous knowledge: novel evidence for major native mammal declines in northern Australia. Biol. Conserv. 157, 78–92. https://doi.org/10.1016/j.biocon.2012.07.004
Media Attributions
- Figure9-1 © Library of Congress Prints and Photographs Division is licensed under a Public Domain license
- Figure9-2 © Brumm et al. adapted by John Lambrinos is licensed under a CC BY-NC (Attribution NonCommercial) license
- Figure9-3 © National Park Service is licensed under a Public Domain license
- Figure9-4 © National Park Service is licensed under a Public Domain license
- Figure9-5 © Jones et al. adapted by John Lambrinos is licensed under a CC BY (Attribution) license
- Figure9-6 © U.S. Geological Survey National Wetlands Research Center; Louisiana Coastal Wetlands Conservation and Restoration Task Force is licensed under a Public Domain license
- Figure9-7 © United States Environmental Protection Agency is licensed under a Public Domain license
- Table9-1a © U.S. Fish and Wildlife Service Northeast Region is licensed under a Public Domain license
- Table9-1b © Doug Smith is licensed under a Public Domain license
- Table9-1c © NOAA
- Table9-1d © IlyaHaykinson is licensed under a CC BY-SA (Attribution ShareAlike) license
- Figure9-8 © Koch et al. is licensed under a Public Domain license
- Figure9-9 © NOAA is licensed under a Public Domain license
- Figure9-10 © Forest and Kim Starr is licensed under a CC BY (Attribution) license
- Figure9-11 © Great Lakes Fishery Commission is licensed under a Public Domain license
- Figure9-12 © González et al. (2020) is licensed under a Public Domain license
- Figure9-13 © R. Trebilco is licensed under a CC BY (Attribution) license
- Figure9-14 © RickP; Openresearch.net adapted by John Lambrinos is licensed under a CC BY-NC-SA (Attribution NonCommercial ShareAlike) license
Blitz, M., 2016. We still don’t know who was first to the North Pole. Popular Mechanics, August 25, 2016.
Icetrek Polar Expeditions, 2019. North Pole and the Arctic. Accessed July 21, 2019, https://icetrek.com/join-a-trip/north-pole-and-arctic.
Aubert, M., Lebe, R., Oktaviana, A.A., Tang, M., Burhan, B., Hamrullah, Jusdi, A., Abdullah, Hakim, B., Zhao, J., Geria, I.M., Sulistyarto, P.H., Sardi, R., Brumm, A., 2019. Earliest hunting scene in prehistoric art. Nature 576, 442–45. https://doi.org/10.1038/s41586-019-1806-y
the attribution of a soul to plants, inanimate objects, and natural phenomena.
Harvey, G., 2014. The Handbook of Contemporary Animism. New York: Routledge.
Haskell, D.G., 2017. The Songs of Trees. New York: Viking.
Catholic Church, 2015. Encyclical letter Laudato Si’ of the Holy Father Francis on care for our common home. May 24, 2015.
Clement, V., 2019. Beyond the sham of the emancipatory enlightenment: rethinking the relationship of Indigenous epistemologies, knowledges, and geography through decolonizing paths. Prog. Human Geogr. 43, 276–94. https://doi.org/10.1177/0309132517747315
Ramos, S.C., 2018. Considerations for culturally sensitive traditional ecological knowledge research in wildlife conservation. Wildl. Soc. Bull. 42, 358–65. https://doi.org/10.1002/wsb.881
Krakauer, J., 1996. Into the Wild. New York: Villard.
Dowie, M., 2009. Conservation Refugees: The Hundred-Year Conflict between Global Conservation and Native Peoples. Cambridge: Massachusetts Institute of Technology Press.
Specia, M., 2019. On Everest, traffic isn’t just inconvenient. It can be deadly. New York Times, May 23, 2019.
Holden, S., 2019. Thailand’s Maya Bay: ‘You think you’re in Times Square’. BBC News, May 10, 2019.
Reyes-Velarde, A., 2019. Instagram-hungry crowds are destroying the super bloom. Los Angeles Times, March 14, 2019.
Ecotourism is about uniting conservation, communities, and sustainable travel. This means that those who implement, participate in and market ecotourism. (From an ecotourism website; a grain of salt is needed.)
Stronza, A.L., Hunt, C.A., Fitzgerald, L.A., 2019. Ecotourism for conservation? Annu. Rev. Environ. Resour. 44, 229-53. https://doi.org/10.1146/annurev-environ-101718-033046
Westphal, M.I., Browne, M., MacKinnon, K., Noble, I., 2008. The link between international trade and the global distribution of invasive alien species. Biol. Invasions 10, 391–98. https://doi.org/10.1007/s10530-007-9138-5
Beaumont, N.J., Aanesen, M., Austen, M.C., Börger, T., Clark, J.R., Cole, M., Hooper, T., Lindeque, P.K., Pascoe, C., Wyles, K.J., 2019. Global ecological, social and economic impacts of marine plastic. Mar. Pollut. Bull. 142, 189–95. https://doi.org/10.1016/j.marpolbul.2019.03.022
Lelieveld, J., Berresheim, H., Borrmann, S., Crutzen, P.J., Dentener, F.J., Fischer, H., Feichter, J., Flatau, P.J., Heland, J., Holzinger, R., Korrmann, R., Lawrence, M.G., Levin, Z., Markowicz, K.M., Mihalopoulos, N., Minikin, A., Ramanathan, V., Reus, M. de, Roelofs, G.J., Scheeren, H.A., Sciare, J., Schlager, H., Schultz, M., Siegmund, P., Steil, B., Stephanou, E.G., Stier, P., Traub, M., Warneke, C., Williams, J., Ziereis, H., 2002. Global air pollution crossroads over the Mediterranean. Science 298, 794–99. https://doi.org/10.1126/science.1075457
Lavoie, R.A., Bouffard, A., Maranger, R., Amyot, M., 2018. Mercury transport and human exposure from global marine fisheries. Sci. Rep. 8, 6705. https://doi.org/10.1038/s41598-018-24938-3
Bradford, W., Morison, S.E., 1952. Of Plymouth Plantation, 1620-1647. New York: Knopf.
Mann, C.C., 2005. 1491: New Revelations of the Americas before Columbus. New York: Knopf.
Winter, G., Fried, 2000. Homeowner perspectives on fire hazard, responsibility, and management strategies at the wildland-urban interface. Soc. Nat. Resour. 13, 33–49. https://doi.org/10.1080/089419200279225
Wilson, E.O., 1984. Biophilia. Cambridge, MA: Harvard University Press.
Loss, S.R., Will, T., Longcore, T., Marra, P.P., 2018. Responding to misinformation and criticisms regarding United States cat predation estimates. Biol. Invasions 20, 3385–96. https://doi.org/10.1007/s10530-018-1796-y
Clements, F.E., 1916. Plant Succession: An Analysis of the Development of Vegetation. Washington, DC: Carnegie Institution.
Ecological succession is the process by which the mix of species and habitat in an area changes over time. For example, after a wildfire, the recolonization of the area takes time and can occur in stages--with each latter stage succeeding the next.
A climax community is the final stage of succession, remaining relatively unchanged until destroyed by an event such as fire or human interference.
Coppoletta, M., Safford, H.D., Estes, B.L., Meyer, M.D., Gross, S.E., Merriam, K.E., Butz, R.J., Molinari, N.A., 2019. Fire regime alteration in natural areas underscores the need to restore a key ecological process. Nat. Areas J. 39, 250–63. https://doi.org/10.3375/043.039.0211
Hall, S.J., Learned, J., Ruddell, B., Larson, K.L., Cavender-Bares, J., Bettez, N., Groffman, P.M., Grove, J.M., Heffernan, J.B., Hobbie, S.E., Morse, J.L., Neill, C., Nelson, K.C., O’Neil-Dunne, J.P.M., Ogden, L., Pataki, D.E., Pearse, W.D., Polsky, C., Chowdhury, R.R., Steele, M.K., Trammell, T.L.E., 2016. Convergence of microclimate in residential landscapes across diverse cities in the United States. Landscape Ecol. 31, 101–17. https://doi.org/10.1007/s10980-015-0297-y
Bachelet, D., Ferschweiler, K., Sheehan, T., Strittholt, J., 2016. Climate change effects on southern California deserts. J. Arid Environ. 127, 17–29. https://doi.org/10.1016/j.jaridenv.2015.10.003
Biological soil crusts (biocrusts) are a complex community of algae, cyanobacteria, lichens, bryophytes, and assorted bacteria, fungi, archaea, and bacteriophages that colonize the soil surface. Biocrusts are particularly common in drylands and are found in arid and semiarid ecosystems worldwide.
Warren, S.D., 2003. Biological soil crusts and hydrology in North American deserts. In: Belnap, J., Lange, O.L. (Eds.), Biological Soil Crusts: Structure, Function, and Management. Ecological Studies. Berlin: Springer, 327–37. https://doi.org/10.1007/978-3-642-56475-8_24
McDonald, C.J., McPherson, G.R., 2011. Fire behavior characteristics of buffelgrass-fueled fires and native plant community composition in invaded patches. J. Arid Environ. 75, 1147–54. https://doi.org/10.1016/j.jaridenv.2011.04.024
Marazzi, B., Bronstein, J.L., Sommers, P.N., López, B.R., Ortega, E.B., Búrquez, A., Medellín, R.A., Aslan, C., Franklin, K., 2015. Plant biotic interactions in the Sonoran Desert: conservation challenges and future directions. J. Southwest 57, 457–501.
Williams, D.G., Baruch, Z., 2000. African grass invasion in the Americas: ecosystem consequences and the role of ecophysiology. Biol. Invasions 2, 123–40. https://doi.org/10.1023/A:1010040524588
A novel ecosystem is a system of abiotic, biotic, and social components (and their interactions) that, by virtue of human influence, differs from those that prevailed historically, having a tendency to self-organize and manifest novel qualities without intensive human interference.
Ecological restoration aims to recreate, initiate, or accelerate the recovery of an ecosystem that has been disturbed. Disturbances are environmental changes that alter ecosystem structure and function. Common disturbances include logging, damming rivers, intense grazing, hurricanes, floods, and fires.
SER. Society for Ecological Restoration, 2019. Mission and vision. Accessed August 2, 2019, https://www.ser.org/page/MissionandVision.
See: restoration ecology (restoration ecology
Peterson, C.H., Anderson, S.S., Cherr, G.N., Ambrose, R.F., Anghera, S., Bay, S., Blum, M., Condon, R., Dean, T.A., Graham, M., Guzy, M., Hampton, S., Joye, S., Lambrinos, J., Mate, B., Meffert, D., Powers, S.P., Somasundaran, P., Spies, R.B., Taylor, C.M., Tjeerdema, R., Adams, E.E., 2012. A tale of two spills: novel science and policy implications of an emerging new oil spill model. BioScience 62, 461–69. https://doi.org/10.1525/bio.2012.62.5.7
Selkoe, K.A., Blenckner, T., Caldwell, M.R., Crowder, L.B., Erickson, A.L., Essington, T.E., Estes, J.A., Fujita, R.M., Halpern, B.S., Hunsicker, M.E., Kappel, C.V., Kelly, R.P., Kittinger, J.N., Levin, P.S., Lynham, J.M., Mach, M.E., Martone, R.G., Mease, L.A., Salomon, A.K., Samhouri, J.F., Scarborough, C., Stier, A.C., White, C., Zedler, J., 2015. Principles for managing marine ecosystems prone to tipping points. Ecosyst. Health Sustainability 1, 1–18. https://doi.org/10.1890/EHS14-0024.1
Poff, N.L., Olden, J.D., Merritt, D.M., Pepin, D.M., 2007. Homogenization of regional river dynamics by dams and global biodiversity implications. Proc. Natl. Acad. Sci. 104, 5732–37. https://doi.org/10.1073/pnas.0609812104
Poff, N.L., Hart, D.D., 2002. How dams vary and why it matters for the emerging science of dam removal. BioScience 52, 659–68. https://doi.org/10.1641/0006-3568(2002)052[0659:HDVAWI]2.0.CO;2
AKA "Shifting Baseline Syndrome", or when the collective environmental knowledge and memory move forward in time with successive generations, resulting in gradual loss of knowledge regarding the previous state of an ecosystem.
Roberts, F., III, 2018. Plaquemines leaders warn about effects of river diversions on fishing industry. NOLA.com, May 22, 2018.
Monsarrat, S., Svenning, J.-C., 2022. Using recent baselines as benchmarks for megafauna restoration places an unfair burden on the Global South. Ecography 2022. https://doi.org/10.1111/ecog.05795
Anderson, K., 2005. Tending the Wild: Native American Knowledge and the Management of California’s Natural Resources. Berkeley: University of California Press.
Lundgren, E.J., Ramp, D., Rowan, J., Middleton, O., Schowanek, S.D., Sanisidro, O., Carroll, S.P., Davis, M., Sandom, C.J., Svenning, J.-C., Wallach, A.D., 2020. Introduced herbivores restore Late Pleistocene ecological functions. Proc. Natl. Acad. Sci. 117, 7871–78. https://doi.org/10.1073/pnas.1915769117
Choi, Y.D., 2007. Restoration ecology to the future: a call for new paradigm. Restor. Ecol. 15, 351–53. https://doi.org/10.1111/j.1526-100X.2007.00224.x
Dumroese, R.K., Williams, M.I., Stanturf, J.A., Clair, J.B.S., 2015. Considerations for restoring temperate forests of tomorrow: forest restoration, assisted migration, and bioengineering. New For. 46, 947–64. https://doi.org/10.1007/s11056-015-9504-6
Ecosystem resilience is the inherent ability to absorb various disturbances and reorganize while undergoing state changes to maintain critical functions. From: Ecological Indicators, 2015.
Mcleod, E., Anthony, K.R.N., Mumby, P.J., Maynard, J., Beeden, R., Graham, N.A.J., Heron, S.F., Hoegh-Guldberg, O., Jupiter, S., MacGowan, P., Mangubhai, S., Marshall, N., Marshall, P.A., McClanahan, T.R., Mcleod, K., Nyström, M., Obura, D., Parker, B., Possingham, H.P., Salm, R.V., Tamelander, J., 2019. The future of resilience-based management in coral reef ecosystems. J. Environ. Manage. 233, 291–301. https://doi.org/10.1016/j.jenvman.2018.11.034
Wildlife corridors are connections across the landscape that link up areas of habitat (see "island theory"). They support natural processes that occur in a healthy environment, including the movement of species to find resources, such as food and water.
Aavik, T., Helm, A., 2018. Restoration of plant species and genetic diversity depends on landscape-scale dispersal. Restor. Ecol. 26, S92–S102. https://doi.org/10.1111/rec.12634
A new concept: areas that form the best route between current climate types and where those climates will occur in the future under climate change.
GIS, or geographic information systems, are computer-based tools used to store, visualize, analyze, and interpret geographic data. Geographic data (also called spatial, or geospatial data) identifies the geographic location of features.
A social-ecological system consists of a "bio-geo-physical" unit and its associated social actors and institutions.
Bird, R.B., Nimmo, D., 2018. Restore the lost ecological functions of people. Nat. Ecol. Evol. 2, 1050–52. https://doi.org/10.1038/s41559-018-0576-5
Jellinek, S., Wilson, K.A., Hagger, V., Mumaw, L., Cooke, B., Guerrero, A.M., Erickson, T.E., Zamin, T., Waryszak, P., Standish, R.J., 2019. Integrating diverse social and ecological motivations to achieve landscape restoration. J. Appl. Ecol. 56, 246–52. https://doi.org/10.1111/1365-2664.13248
Jones, H.P., Jones, P.C., Barbier, E.B., Blackburn, R.C., Rey, B.J.M., Holl, K.D., McCrackin, M., Meli, P., Montoya, D., Mateos, D.M., 2018. Restoration and repair of Earth’s damaged ecosystems. Proc. R. Soc. B Biol. Sci. 285, 20172577. https://doi.org/10.1098/rspb.2017.2577
Larkin, D.J., Buck, R.J., Fieberg, J., Galatowitsch, S.M., 2019. Revisiting the benefits of active approaches for restoring damaged ecosystems. A comment on Jones HP et al. 2018, Restoration and repair of Earth’s damaged ecosystems. Proc. R. Soc. B Biol. Sci. 286, 20182928. https://doi.org/10.1098/rspb.2018.2928
Lindh, B.C., 2018. Tipping the native-exotic balance: succession in restored upland prairies in Oregon’s Willamette Valley. Ecol. Restor. 36, 28–40. https://doi.org/10.3368/er.36.1.28
An ecotype is a population (or subspecies or race) that is adapted to local environmental conditions. Evolutionary biologist and ecologists mean to imply that said populations were those best suited and survived.
Vander Mijnsbrugge, K., Bischoff, A., Smith, B., 2010. A question of origin: where and how to collect seed for ecological restoration. Basic Appl. Ecol. 11, 300–311. https://doi.org/10.1016/j.baae.2009.09.002
Larkin, D., Vivian-Smith, G., Zedler, Joy B., 2013. Topographic heterogeneity theory and ecological restoration. In: Falk, D.A., Palmer, M.A., Zedler, J.B. (Eds.), Foundations of Restoration Ecology. Washington, DC: Island Press.
Diefenderfer, H.L., Sinks, I.A., Zimmerman, S.A., Cullinan, V.I., Borde, A.B., 2018. Designing topographic heterogeneity for tidal wetland restoration. Ecol. Eng. 123, 212–25. https://doi.org/10.1016/j.ecoleng.2018.07.027
McCallum, K.P., Lowe, A.J., Breed, M.F., Paton, D.C., 2018. Spatially designed revegetation—why the spatial arrangement of plants should be as important to revegetation as they are to natural systems. Restor. Ecol. 26, 446–55. https://doi.org/10.1111/rec.12690
An organism whose presence, absence or abundance reflects a specific environmental condition.
Plummer, M.L., Harvey, C.J., Anderson, L.E., Guerry, A.D., Ruckelshaus, M.H., 2013. The Role of eelgrass in marine community interactions and ecosystem services: results from ecosystem-scale food web models. Ecosystems 16, 237–51. https://doi.org/10.1007/s10021-012-9609-0
Short, F.T., Burdick, D.M., Short, C.A., Davis, R.C., Morgan, P.A., 2000. Developing success criteria for restored eelgrass, salt marsh and mud flat habitats. Ecol. Eng. 15, 239–52. https://doi.org/10.1016/S0925-8574(00)00079-3
Paine, R.T., Ruesink, J.L., Sun, A., Soulanille, E.L., Wonham, M.J., Harley, C.D.G., Brumbaugh, D.R., Secord, D.L., 1996. Trouble on oiled waters: lessons from the Exxon Valdez oil spill. Annu. Rev. Ecol. Syst. 27, 197–235. https://doi.org/10.1146/annurev.ecolsys.27.1.197
Esler, D., Ballachey, B.E., Matkin, C., Cushing, D., Kaler, R., Bodkin, J., Monson, D., Esslinger, G., Kloecker, K., 2018. Timelines and mechanisms of wildlife population recovery following the Exxon Valdez oil spill. In: Aderhold, D.R., Esler, D., Heintz, R.A., Hopcroft, R.R., Lindeberg, M.R., Pegau, W.S. (Eds.), Spatial and temporal ecological variability in the northern gulf of Alaska: What have we learned since the Exxon Valdez oil spill?, Special issue, Deep Sea Res. Part II Top. Stud. Oceanogr., 147, 36–42. https://doi.org/10.1016/j.dsr2.2017.04.007
Silliman, B.R., Koppel, J. van de, McCoy, M.W., Diller, J., Kasozi, G.N., Earl, K., Adams, P.N., Zimmerman, A.R., 2012. Degradation and resilience in Louisiana salt marshes after the BP–Deepwater Horizon oil spill. Proc. Natl. Acad. Sci. 109, 11,234–39. https://doi.org/10.1073/pnas.1204922109
Ballew, N.G., Bacheler, N.M., Kellison, G.T., Schueller, A.M., 2016. Invasive lionfish reduce native fish abundance on a regional scale. Sci. Rep. 6, 32169. https://doi.org/10.1038/srep32169
Fusco, E.J., Finn, J.T., Balch, J.K., Nagy, R.C., Bradley, B.A., 2019. Invasive grasses increase fire occurrence and frequency across US ecoregions. Proc. Natl. Acad. Sci. 116, 23,594-99. https://doi.org/10.1073/pnas.1908253116
Enright, W.D., 2000. The effect of terrestrial invasive alien plants on water scarcity in South Africa. Phys. Chem. Earth Part B Hydrol. Oceans Atmos. 25, 237–42. https://doi.org/10.1016/S1464-1909(00)00010-1
Paini, D.R., Sheppard, A.W., Cook, D.C., Barro, P.J.D., Worner, S.P., Thomas, M.B., 2016. Global threat to agriculture from invasive species. Proc. Natl. Acad. Sci. 113, 7575–79. https://doi.org/10.1073/pnas.1602205113
Mazza, G., Tricarico, E., 2018. Invasive Species and Human Health. Wallingford, UK: CABI.
Pratt, C.F., Constantine, K.L., Murphy, S.T., 2017. Economic impacts of invasive alien species on African smallholder livelihoods. In: Pérez-Escamilla, R., Levy, T.S. (Eds.), Food Security Governance in Latin America, special issue, Global Food Secur. 14, 31–37. https://doi.org/10.1016/j.gfs.2017.01.011
Nunez‐Mir, G. C., Guo, Q., Rejmánek, M., Iannone, B. V., & Fei, S. 2019. Predicting invasiveness of exotic woody species using a traits-based framework. Ecology, 100(10), e02797. https://doi.org/10.1002/ecy.2797
Ward, S.F., Fei, S., Liebhold, A.M., 2020. Temporal dynamics and drivers of landscape-level spread by emerald ash borer. J. Appl. Ecol. 57, 1020–30. https://doi.org/10.1111/1365-2664.13613
Banks, N.C., Paini, D.R., Bayliss, K.L., Hodda, M., 2015. The role of global trade and transport network topology in the human-mediated dispersal of alien species. Ecol. Lett. 18, 188–99. https://doi.org/10.1111/ele.12397
Seebens, H., Blackburn, T.M., Dyer, E.E., Genovesi, P., Hulme, P.E., Jeschke, J.M., Pagad, S., Pyšek, P., Winter, M., Arianoutsou, M., Bacher, S., Blasius, B., Brundu, G., Capinha, C., Celesti-Grapow, L., Dawson, W., Dullinger, S., Fuentes, N., Jäger, H., Kartesz, J., Kenis, M., Kreft, H., Kühn, I., Lenzner, B., Liebhold, A., Mosena, A., Moser, D., Nishino, M., Pearman, D., Pergl, J., Rabitsch, W., Rojas-Sandoval, J., Roques, A., Rorke, S., Rossinelli, S., Roy, H.E., Scalera, R., Schindler, S., Štajerová, K., Tokarska-Guzik, B., van Kleunen, M., Walker, K., Weigelt, P., Yamanaka, T., Essl, F., 2017. No saturation in the accumulation of alien species worldwide. Nat. Commun. 8, ncomms14435. https://doi.org/10.1038/ncomms14435
Hulme, P.E., Brundu, G., Carboni, M., Dehnen‐Schmutz, K., Dullinger, S., Early, R., Essl, F., González‐Moreno, P., Groom, Q.J., Kueffer, C., Kühn, I., Maurel, N., Novoa, A., Pergl, J., Pyšek, P., Seebens, H., Tanner, R., Touza, J.M., van Kleunen, M., Verbrugge, L.N.H., 2018. Integrating invasive species policies across ornamental horticulture supply chains to prevent plant invasions. J. Appl. Ecol. 55, 92–98. https://doi.org/10.1111/1365-2664.12953
CABI, 2019. Datasheet for Acer platanoides (Norway maple). In: Invasive Species Compendium. Wallingford, UK: CAB International. www.cabi.org/isc.
Conklin, J.R., Sellmer, J.C., 2009. Flower and seed production of Norway maple cultivars. HortTechnology 19, 91–95. https://doi.org/10.21273/HORTSCI.19.1.91
Vining, K.J., Contreras, R.N., Ranik, M., Strauss, S.H., 2012. Genetic methods for mitigating invasiveness of woody ornamental plants: research needs and opportunities. HortScience 47, 1210–16. https://doi.org/10.21273/HORTSCI.47.9.1210
The Enemy Release Hypothesis (ERH) predicts that a species will be successful in a new habitat when former predators are not present. Example: Himalayan blackberries, ash borer.
Courchamp, F., Chapuis, J.-L., Pascal, M., 2003. Mammal invaders on islands: impact, control and control impact. Biol. Rev. 78, 347–83. https://doi.org/10.1017/S1464793102006061
Aarde, R.J.V., 1980. The diet and feeding behaviour of feral cats, Felis catus at Marion Island. S. Afr. Tydskr. Natuurnav. 10, 123–28.
Asplen, M.K., Anfora, G., Biondi, A., Choi, D.-S., Chu, D., Daane, K.M., Gibert, P., Gutierrez, A.P., Hoelmer, K.A., Hutchison, W.D., Isaacs, R., Jiang, Z.-L., Kárpáti, Z., Kimura, M.T., Pascual, M., Philips, C.R., Plantamp, C., Ponti, L., Vétek, G., Vogt, H., Walton, V.M., Yu, Y., Zappalà, L., Desneux, N., 2015. Invasion biology of spotted wing Drosophila (Drosophila suzukii): a global perspective and future priorities. J. Pest Sci. 88, 469–94. https://doi.org/10.1007/s10340-015-0681-z
Klick, J., Rodriguez-Saona, C.R., Cumplido, J.H., Holdcraft, R.J., Urrutia, W.H., da Silva, R.O., Borges, R., Mafra-Neto, A., Seagraves, M.P., 2019. Testing a novel attract-and-kill strategy for Drosophila suzukii (Diptera: Drosophilidae) management. J. Insect Sci. 19. https://doi.org/10.1093/jisesa/iey132
Biological control is the use of living organisms to suppress pest populations, making them less damaging than they would otherwise be. Natural enemies of insects play an important role in limiting the densities of potential pests. For example: in America, gardeners often employ the ladybird/ladybug to great effect.
Hoddle, M.S., 2004. Restoring balance: using exotic species to control invasive exotic species. Conserv. Biol. 18, 38–49. https://doi.org/10.1111/j.1523-1739.2004.00249.x
Hinz, H.L., Winston, R.L., Schwarzländer, M., 2019. How safe is weed biological control? A global review of direct nontarget attack. Q. Rev. Biol. 94, 1–27. https://doi.org/10.1086/702340
Lyons, K.G., Maldonado-Leal, B.G., Owen, G., 2013. Community and ecosystem effects of buffelgrass (Pennisetum ciliare) and nitrogen deposition in the Sonoran Desert. Invasive Plant Sci. Manage. 6, 65–78. https://doi.org/10.1614/IPSM-D-11-00071.1
Szili-Kovács, T., Török, K., Tilston, E.L., Hopkins, D.W., 2007. Promoting microbial immobilization of soil nitrogen during restoration of abandoned agricultural fields by organic additions. Biol. Fertil. Soils 43, 823–28. https://doi.org/10.1007/s00374-007-0182-1
Ziembicki, M.R., Woinarski, J.C.Z., Mackey, B., 2013. Evaluating the status of species using Indigenous knowledge: novel evidence for major native mammal declines in northern Australia. Biol. Conserv. 157, 78–92. https://doi.org/10.1016/j.biocon.2012.07.004
Woinarski, J.C.Z., Burbidge, A.A., Harrison, P.L., 2015. Ongoing unraveling of a continental fauna: decline and extinction of Australian mammals since European settlement. Proc. Natl. Acad. Sci. 112, 4531–40. https://doi.org/10.1073/pnas.1417301112
Legge, S., Woinarski, J.C.Z., Burbidge, A.A., Palmer, R., Ringma, J., Radford, J.Q., Mitchell, N., Bode, M., Wintle, B., Baseler, M., Bentley, J., Copley, P., Dexter, N., Dickman, C.R., Gillespie, G.R., Hill, B., Johnson, C.N., Latch, P., Letnic, M., Manning, A., McCreless, E.E., Menkhorst, P., Morris, K., Moseby, K., Page, M., Pannell, D., Tuft, K., 2018. Havens for threatened Australian mammals: the contributions of fenced areas and offshore islands to the protection of mammal species susceptible to introduced predators. Wildl. Res. 45, 627–44. https://doi.org/10.1071/WR17172
Predator Free 2050 Limited, 2022. Home page. Accessed May 22, 2022, https://pf2050.co.nz/.
Russell, J.C., Innes, J.G., Brown, P.H., Byrom, A.E., 2015. Predator-free New Zealand: conservation country. BioScience 65, 520–25. https://doi.org/10.1093/biosci/biv012
Miehls, S., Sullivan, P., Twohey, M., Barber, J., McDonald, R., 2020. The future of barriers and trapping methods in the sea lamprey (Petromyzon marinus) control program in the Laurentian Great Lakes. Rev. Fish Biol. Fish. 30, 1–24. https://doi.org/10.1007/s11160-019-09587-7
Great Lakes Fishery Commission, 2020. Budget. Accessed July 23, 2020, http://www.glfc.org/budget.php.
Wilkie, M.P., Hubert, T.D., Boogaard, M.A., Birceanu, O., 2019. Control of invasive sea lampreys using the piscicides TFM and niclosamide: toxicology, successes and future prospects. Aquat. Toxicol. 211, 235–52. https://doi.org/10.1016/j.aquatox.2018.12.012
Siefkes, M.J., 2017. Use of physiological knowledge to control the invasive sea lamprey (Petromyzon marinus) in the Laurentian Great Lakes. Conserv. Physiol. 5. https://doi.org/10.1093/conphys/cox031
A population of hybrids that has survived beyond the initial hybrid generation, with interbreeding between hybrid individuals and backcrossing with its parent types. Consider: attempts at crossbreeding European and African honeybees; most modern rose varieties.
Dudley, T.L., DeLoach, C.J., Levich, J.E., Carruthers, R.I., 2000. Saltcedar invasion of western riparian areas: impacts and new prospects for control. In: Transactions of the 65th North American Wildlife and Natural Resources Conference , Rosemont, Illinois. Wildlife Management Institute, Washington, D.C., pp. 345–381.
Merritt, D.M., Poff, N.L.R., 2010. Shifting dominance of riparian Populus and Tamarix along gradients of flow alteration in western North American rivers. Ecol. Appl. 20, 135–52. https://doi.org/10.1890/08-2251.1
Bean, D.W., Dalin, P., Dudley, T.L., 2012. Evolution of critical day length for diapause induction enables range expansion of Diorhabda carinulata, a biological control agent against tamarisk (Tamarix spp.). Evol. Appl. 5, 511–23. https://doi.org/10.1111/j.1752-4571.2012.00262.x
A period of suspended development in an insect, other invertebrate, or mammal embryo, especially during unfavorable environmental conditions
Bean, D., Dudley, T., 2018. A synoptic review of Tamarix biocontrol in North America: tracking success in the midst of controversy. BioControl 63, 361–76. https://doi.org/10.1007/s10526-018-9880-x
González, E., Shafroth, P.B., Lee, S.R., Ostoja, S.M., Brooks, M.L., 2020. Combined effects of biological control of an invasive shrub and fluvial processes on riparian vegetation dynamics. Biol. Invasions 22, 2339–56. https://doi.org/10.1007/s10530-020-02259-9
Cleeland, J.B., Pardo, D., Raymond, B., Terauds, A., Alderman, R., McMahon, C.R., Phillips, R.A., Lea, M.-A., Hindell, M.A., 2020. Introduced species and extreme weather as key drivers of reproductive output in three sympatric albatrosses. Sci. Rep. 10, 8199. https://doi.org/10.1038/s41598-020-64662-5
Bergstrom, D.M., Lucieer, A., Kiefer, K., Wasley, J., Belbin, L., Pedersen, T.K., Chown, S.L., 2009. Indirect effects of invasive species removal devastate World Heritage Island. J. Appl. Ecol. 46, 73–81. https://doi.org/10.1111/j.1365-2664.2008.01601.x
Dowding, J.E., Murphy, E.C., Springer, K., Peacock, A.J., Krebs, C.J., 2009. Cats, rabbits, Myxoma virus, and vegetation on Macquarie Island: a comment on Bergstrom et al. (2009). J. Appl. Ecol. 46, 1129–32. https://doi.org/10.1111/j.1365-2664.2009.01690.x
Springer, K., 2018. Eradication of invasive species on Macquarie Island to restore the natural ecosystem. In: Recovering Australian Threatened Species: A Book of Hope. Clayton, Australia: CSIRO.
Flory, S.L., D’Antonio, C.M., 2015. Taking the long view on the ecological effects of plant invasions. Am. J. Bot. 102, 817–18. https://doi.org/10.3732/ajb.1500105
Cassini, M.H., 2020. A review of the critics of invasion biology. Biol. Rev. 95, 1467-78. https://doi.org/10.1111/brv.12624
Chew, M.K., Hamilton, A. L, 2011. Chew, M. K., & Hamilton, A. L. 2011. The rise and fall of biotic nativeness: a historical perspective. In: Fifty Years of Invasion Ecology: The Legacy of Charles Elton. New York: John Wiley & Sons.
Bhattacharyya, J., Slocombe, D.S., Murphy, S.D., 2011. The “wild” or “feral” distraction: effects of cultural understandings on management controversy over free-ranging horses (Equus ferus caballus). Human Ecol. 39, 613–25. https://doi.org/10.1007/s10745-011-9416-9
Davis, M.A., Chew, M.K., Hobbs, R.J., Lugo, A.E., Ewel, J.J., Vermeij, G.J., Brown, J.H., Rosenzweig, M.L., Gardener, M.R., Carroll, S.P., Thompson, K., Pickett, S.T.A., Stromberg, J.C., Tredici, P.D., Suding, K.N., Ehrenfeld, J.G., Philip Grime, J., Mascaro, J., Briggs, J.C., 2011. Don’t judge species on their origins. Nature 474, 153–54. https://doi.org/10.1038/474153a
Chew, M.K., 2015. Ecologists, environmentalists, experts, and the invasion of the second greatest threat. Int. Rev. Environ. Hist. 1, 7–40.
Marris, E., 2013. Rambunctious Garden: Saving Nature in a Post-Wild World. London: Bloomsbury.
Pauchard, A., Meyerson, L.A., Bacher, S., Blackburn, T.M., Brundu, G., Cadotte, M.W., Courchamp, F., Essl, F., Genovesi, P., Haider, S., Holmes, N.D., Hulme, P.E., Jeschke, J.M., Lockwood, J.L., Novoa, A., Nuñez, M.A., Peltzer, D.A., Pyšek, P., Richardson, D.M., Simberloff, D., Smith, K., Wilgen, B.W. van, Vilà, M., Wilson, J.R.U., Winter, M., Zenni, R.D., 2018. Biodiversity assessments: origin matters. PLoS Biol. 16, e2006686. https://doi.org/10.1371/journal.pbio.2006686
Crystal-Ornelas, R., Lockwood, J.L., 2020. The “known unknowns” of invasive species impact measurement. Biol. Invasions 22, 1513–25. https://doi.org/10.1007/s10530-020-02200-0
Russell, J.C., Blackburn, T.M., 2017. The rise of invasive species denialism. Trends Ecol. Evol. 32, 3–6. https://doi.org/10.1016/j.tree.2016.10.012
Carroll, S.P., Klassen, S.P., Dingle, H., 1998. Rapidly evolving adaptations to host ecology and nutrition in the soapberry bug. Evol. Ecol. 12, 955–68. https://doi.org/10.1023/A:1006568206413
Zavaleta, E.S., Hobbs, R.J., Mooney, H.A., 2001. Viewing invasive species removal in a whole-ecosystem context. Trends Ecol. Evol. 16, 454–59. https://doi.org/10.1016/S0169-5347(01)02194-2
Prior, K.M., Adams, D.C., Klepzig, K.D., Hulcr, J., 2018. When does invasive species removal lead to ecological recovery? Implications for management success. Biol. Invasions 20, 267–83. https://doi.org/10.1007/s10530-017-1542-x
Crowley, S.L., Hinchliffe, S., McDonald, R.A., 2017. Conflict in invasive species management. Front. Ecol. Environ. 15, 133–41. https://doi.org/10.1002/fee.1471
Carroll, S.P., 2011. Conciliation biology: the eco-evolutionary management of permanently invaded biotic systems. Evol. Appl. 4, 184–99. https://doi.org/10.1111/j.1752-4571.2010.00180.x
Kueffer, C., Kull, C.A., 2017. Non-native species and the aesthetics of nature. In: Vilà, M., Hulme, P.E. (Eds.), Impact of Biological Invasions on Ecosystem Services. Invading Nature: Springer Series in Invasion Ecology. Cham, Switzerland: Springer International, 311–24. https://doi.org/10.1007/978-3-319-45121-3_20
Bhattacharyya, J., Larson, B.M.H., 2014. The need for Indigenous voices in discourse about introduced species: insights from a controversy over wild horses. Environ. Values 23, 663–84. https://doi.org/10.3197/096327114X13947900181031
Kueffer, C., Kaiser-Bunbury, C.N., 2014. Reconciling conflicting perspectives for biodiversity conservation in the Anthropocene. Front. Ecol. Environ. 12, 131–37. https://doi.org/10.1890/120201
Funk, J.L., Matzek, V., Bernhardt, M., Johnson, D., 2014. Broadening the case for invasive species management to include impacts on ecosystem services. BioScience 64, 58–63. https://doi.org/10.1093/biosci/bit004
Alexander, J.M., Frankel, S.J., Hapner, N., Phillips, J.L., Dupuis, V., 2017. Working across cultures to protect Native American natural and cultural resources from invasive species in California. J. For. 115, 473–79. https://doi.org/10.5849/jof.16-018
Bekoff, M., 2010. The Animal Manifesto: Six Reasons for Expanding Our Compassion Footprint. Novato, CA: New World Library.
Gruenberg, B.U., 2016. The Wild Horse Dilemma: Conflicts and Controversies of the Atlantic Coast Herds. New Providence, PA: Synclitic Media.
Sandler, R.L., Sandler, R.L., 2012. The Ethics of Species: An Introduction. Cambridge: Cambridge University Press.
Wallach, A.D., Bekoff, M., Batavia, C., Nelson, M.P., Ramp, D., 2018. Summoning compassion to address the challenges of conservation. Conserv. Biol. 32, 1255–65. https://doi.org/10.1111/cobi.13126
Hayward, M.W., Callen, A., Allen, B.L., Ballard, G., Broekhuis, F., Bugir, C., Clarke, R.H., Clulow, J., Clulow, S., Daltry, J.C., Davies‐Mostert, H.T., Fleming, P.J.S., Griffin, A.S., Howell, L.G., Kerley, G.I.H., Klop‐Toker, K., Legge, S., Major, T., Meyer, N., Montgomery, R.A., Moseby, K., Parker, D.M., Périquet, S., Read, J., Scanlon, R.J., Seeto, R., Shuttleworth, C., Somers, M.J., Tamessar, C.T., Tuft, K., Upton, R., Valenzuela‐Molina, M., Wayne, A., Witt, R.R., Wüster, W., 2019. Deconstructing compassionate conservation. Conserv. Biol. 33, 760–68. https://doi.org/10.1111/cobi.13366
Teem, J.L., Alphey, L., Descamps, S., Edgington, M.P., Edwards, O., Gemmell, N., Harvey-Samuel, T., Melnick, R.L., Oh, K.P., Piaggio, A.J., Saah, J.R., Schill, D., Thomas, P., Smith, T., Roberts, A., 2020. Genetic biocontrol for invasive species. Front. Bioeng. Biotechnol. 8. https://doi.org/10.3389/fbioe.2020.00452
Colautti, R.I., MacIsaac, H.J., 2004. A neutral terminology to define “invasive” species. Diversity Distrib. 10, 135–41. https://doi.org/10.1111/j.1366-9516.2004.00061.x
Shackleton, R.T., Larson, B.M.H., Novoa, A., Richardson, D.M., Kull, C.A., 2019. The human and social dimensions of invasion science and management. In: Shackleton, R.T., Larson, B.M.H., Novoa, A., Richardson, D.M., Kull, C.A. (Eds.), The Human and Social Dimensions of Invasion Science and Management, special issue, J. Environ. Manage. 229, 1–9. https://doi.org/10.1016/j.jenvman.2018.08.041
Shackleton, R.T., Witt, A.B., Aool, W., Pratt, C.F., 2017. Distribution of the invasive alien weed, Lantana camara, and its ecological and livelihood impacts in eastern Africa. Afr. J. Range Forage Sci. 34, 1–11. https://doi.org/10.2989/10220119.2017.1301551
Kannan, R., Shackleton, C.M., Shaanker, R.U., 2014. Invasive alien species as drivers in socio-ecological systems: local adaptations towards use of Lantana in Southern India. Environ. Dev. Sustainability 16, 649–69. https://doi.org/10.1007/s10668-013-9500-y
Caceres‐Escobar, H., Kark, S., Atkinson, S.C., Possingham, H.P., Davis, K.J., 2019. Integrating local knowledge to prioritise invasive species management. People Nat. 1, 220–33. https://doi.org/10.1002/pan3.27
A branch of invasive-study biology that is concerned with managing long-term interactions between native and non-native species. Conciliation biology specifically does not concern itself with elimination or removal, but instead with the longer-term outcomes of native-non-native interactions at the individual, population, species, community, and ecosystem levels.